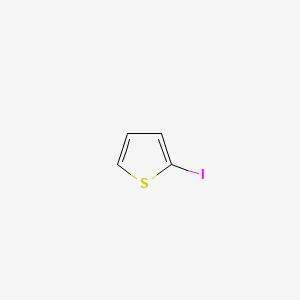
2-Iodothiophene
Descripción general
Descripción
2-Iodothiophene is a colorless to light yellow liquid . It has been used in the synthesis of (E)-and (Z)-2,3-difluoro-3-aryl substituted acrylic esters. It was also used as a monomer in the preparation of thin conductive films via plasma polymerization of 2-iodothiophene .
Synthesis Analysis
2-Iodothiophene can be synthesized by a fluoride-free, palladium-catalyzed cross-coupling reaction with potassium (E)-heptenyldimethylsilanolate . Another approach employs photocatalytic decarboxylative iodination of thiophene 2-carboxylic acid . Nickel-catalyzed bromine to iodine exchange of 2-bromothiophene can also be used .
Molecular Structure Analysis
The molecular formula of 2-Iodothiophene is C4H3IS . The molecular weight is 210.04 g/mol . The IUPAC Standard InChI is InChI=1S/C4H3IS/c5-4-2-1-3-6-4/h1-3H .
Chemical Reactions Analysis
2-Iodothiophene participates in microwave-enhanced, rapid homogeneous-phase version of the Sonogashira reaction . It has been used in the synthesis of (E)-and (Z)-2,3-difluoro-3-aryl substituted acrylic esters .
Physical And Chemical Properties Analysis
2-Iodothiophene has a molecular weight of 210.04 g/mol . It has a topological polar surface area of 28.2 Ų . The complexity of 2-Iodothiophene is 46.8 .
Aplicaciones Científicas De Investigación
Synthesis of Thiophene Derivatives
2-Iodothiophene plays a crucial role in the synthesis of thiophene derivatives . Thiophene-based analogs are of great interest to scientists as they are a potential class of biologically active compounds . They are used by medicinal chemists to develop advanced compounds with a variety of biological effects .
Industrial Chemistry and Material Science
Thiophene derivatives, including 2-Iodothiophene, are utilized in industrial chemistry and material science as corrosion inhibitors . They are essential heterocyclic compounds and show a variety of properties and applications .
Organic Semiconductors
2-Iodothiophene-mediated molecules play a significant role in the advancement of organic semiconductors . They are used in the development of organic field-effect transistors (OFETs) and in the fabrication
Mecanismo De Acción
Target of Action
2-Iodothiophene is a derivative of thiophene, a five-membered heterocyclic compound with a sulfur atom . Thiophene derivatives have been recognized for their potential as biologically active compounds . They play a crucial role in medicinal chemistry, contributing to the development of advanced compounds with a variety of biological effects . .
Result of Action
It’s known that the uv photochemistry of 2-iodothiophene serves as a testbed for understanding fundamental photo-induced chemical transformations in moderately complex compounds, including isomerization, ring-opening, and molecular dissociation .
Action Environment
The action of 2-Iodothiophene is influenced by environmental factors such as light. For instance, UV light-induced dynamics in 2-Iodothiophene have been studied . .
Safety and Hazards
Direcciones Futuras
A combined experimental-theoretical study of 268 nm UV light-induced dynamics in 2-iodothiophene (C4H3IS) has been performed . The dynamics were experimentally monitored with a femtosecond extreme ultraviolet (XUV) probe that measures iodine N-edge 4d core-to-valence transitions . This research serves as a testbed for understanding fundamental photoinduced transformations in moderately complex compounds, including isomerization, ring-opening, and molecular dissociation .
Propiedades
IUPAC Name |
2-iodothiophene | |
---|---|---|
Source | PubChem | |
URL | https://pubchem.ncbi.nlm.nih.gov | |
Description | Data deposited in or computed by PubChem | |
InChI |
InChI=1S/C4H3IS/c5-4-2-1-3-6-4/h1-3H | |
Source | PubChem | |
URL | https://pubchem.ncbi.nlm.nih.gov | |
Description | Data deposited in or computed by PubChem | |
InChI Key |
ROIMNSWDOJCBFR-UHFFFAOYSA-N | |
Source | PubChem | |
URL | https://pubchem.ncbi.nlm.nih.gov | |
Description | Data deposited in or computed by PubChem | |
Canonical SMILES |
C1=CSC(=C1)I | |
Source | PubChem | |
URL | https://pubchem.ncbi.nlm.nih.gov | |
Description | Data deposited in or computed by PubChem | |
Molecular Formula |
C4H3IS | |
Source | PubChem | |
URL | https://pubchem.ncbi.nlm.nih.gov | |
Description | Data deposited in or computed by PubChem | |
DSSTOX Substance ID |
DTXSID0063026 | |
Record name | Thiophene, 2-iodo- | |
Source | EPA DSSTox | |
URL | https://comptox.epa.gov/dashboard/DTXSID0063026 | |
Description | DSSTox provides a high quality public chemistry resource for supporting improved predictive toxicology. | |
Molecular Weight |
210.04 g/mol | |
Source | PubChem | |
URL | https://pubchem.ncbi.nlm.nih.gov | |
Description | Data deposited in or computed by PubChem | |
Product Name |
2-Iodothiophene | |
CAS RN |
3437-95-4 | |
Record name | 2-Iodothiophene | |
Source | CAS Common Chemistry | |
URL | https://commonchemistry.cas.org/detail?cas_rn=3437-95-4 | |
Description | CAS Common Chemistry is an open community resource for accessing chemical information. Nearly 500,000 chemical substances from CAS REGISTRY cover areas of community interest, including common and frequently regulated chemicals, and those relevant to high school and undergraduate chemistry classes. This chemical information, curated by our expert scientists, is provided in alignment with our mission as a division of the American Chemical Society. | |
Explanation | The data from CAS Common Chemistry is provided under a CC-BY-NC 4.0 license, unless otherwise stated. | |
Record name | 2-Iodothiophene | |
Source | ChemIDplus | |
URL | https://pubchem.ncbi.nlm.nih.gov/substance/?source=chemidplus&sourceid=0003437954 | |
Description | ChemIDplus is a free, web search system that provides access to the structure and nomenclature authority files used for the identification of chemical substances cited in National Library of Medicine (NLM) databases, including the TOXNET system. | |
Record name | 2-IODOTHIOPHENE | |
Source | DTP/NCI | |
URL | https://dtp.cancer.gov/dtpstandard/servlet/dwindex?searchtype=NSC&outputformat=html&searchlist=1082 | |
Description | The NCI Development Therapeutics Program (DTP) provides services and resources to the academic and private-sector research communities worldwide to facilitate the discovery and development of new cancer therapeutic agents. | |
Explanation | Unless otherwise indicated, all text within NCI products is free of copyright and may be reused without our permission. Credit the National Cancer Institute as the source. | |
Record name | Thiophene, 2-iodo- | |
Source | EPA Chemicals under the TSCA | |
URL | https://www.epa.gov/chemicals-under-tsca | |
Description | EPA Chemicals under the Toxic Substances Control Act (TSCA) collection contains information on chemicals and their regulations under TSCA, including non-confidential content from the TSCA Chemical Substance Inventory and Chemical Data Reporting. | |
Record name | Thiophene, 2-iodo- | |
Source | EPA DSSTox | |
URL | https://comptox.epa.gov/dashboard/DTXSID0063026 | |
Description | DSSTox provides a high quality public chemistry resource for supporting improved predictive toxicology. | |
Record name | 2-iodothiophene | |
Source | European Chemicals Agency (ECHA) | |
URL | https://echa.europa.eu/substance-information/-/substanceinfo/100.020.312 | |
Description | The European Chemicals Agency (ECHA) is an agency of the European Union which is the driving force among regulatory authorities in implementing the EU's groundbreaking chemicals legislation for the benefit of human health and the environment as well as for innovation and competitiveness. | |
Explanation | Use of the information, documents and data from the ECHA website is subject to the terms and conditions of this Legal Notice, and subject to other binding limitations provided for under applicable law, the information, documents and data made available on the ECHA website may be reproduced, distributed and/or used, totally or in part, for non-commercial purposes provided that ECHA is acknowledged as the source: "Source: European Chemicals Agency, http://echa.europa.eu/". Such acknowledgement must be included in each copy of the material. ECHA permits and encourages organisations and individuals to create links to the ECHA website under the following cumulative conditions: Links can only be made to webpages that provide a link to the Legal Notice page. | |
Record name | 2-Iodothiophene | |
Source | FDA Global Substance Registration System (GSRS) | |
URL | https://gsrs.ncats.nih.gov/ginas/app/beta/substances/Z4KJ38KVA6 | |
Description | The FDA Global Substance Registration System (GSRS) enables the efficient and accurate exchange of information on what substances are in regulated products. Instead of relying on names, which vary across regulatory domains, countries, and regions, the GSRS knowledge base makes it possible for substances to be defined by standardized, scientific descriptions. | |
Explanation | Unless otherwise noted, the contents of the FDA website (www.fda.gov), both text and graphics, are not copyrighted. They are in the public domain and may be republished, reprinted and otherwise used freely by anyone without the need to obtain permission from FDA. Credit to the U.S. Food and Drug Administration as the source is appreciated but not required. | |
Retrosynthesis Analysis
AI-Powered Synthesis Planning: Our tool employs the Template_relevance Pistachio, Template_relevance Bkms_metabolic, Template_relevance Pistachio_ringbreaker, Template_relevance Reaxys, Template_relevance Reaxys_biocatalysis model, leveraging a vast database of chemical reactions to predict feasible synthetic routes.
One-Step Synthesis Focus: Specifically designed for one-step synthesis, it provides concise and direct routes for your target compounds, streamlining the synthesis process.
Accurate Predictions: Utilizing the extensive PISTACHIO, BKMS_METABOLIC, PISTACHIO_RINGBREAKER, REAXYS, REAXYS_BIOCATALYSIS database, our tool offers high-accuracy predictions, reflecting the latest in chemical research and data.
Strategy Settings
Precursor scoring | Relevance Heuristic |
---|---|
Min. plausibility | 0.01 |
Model | Template_relevance |
Template Set | Pistachio/Bkms_metabolic/Pistachio_ringbreaker/Reaxys/Reaxys_biocatalysis |
Top-N result to add to graph | 6 |
Feasible Synthetic Routes
Q & A
Q1: What is the molecular formula and weight of 2-iodothiophene?
A1: 2-Iodothiophene has the molecular formula C4H3IS and a molecular weight of 210.06 g/mol.
Q2: What spectroscopic data is available for characterizing 2-iodothiophene?
A2: Researchers commonly use techniques like Nuclear Magnetic Resonance (NMR) spectroscopy, Infrared (IR) spectroscopy, and mass spectrometry (MS) to characterize 2-iodothiophene. [] Additionally, studies have investigated its valence shell photoelectron spectrum using synchrotron radiation and high-resolution photoelectron spectroscopy. [, ] These techniques provide insights into the compound's structure, bonding, and electronic properties.
Q3: How is 2-iodothiophene utilized in cross-coupling reactions?
A3: 2-Iodothiophene serves as a valuable substrate in palladium-catalyzed cross-coupling reactions, such as the Sonogashira reaction. [, ] It reacts with terminal alkynes in the presence of a palladium catalyst and a copper co-catalyst to form substituted alkynes. This reaction is particularly useful for synthesizing conjugated polymers and organic semiconductors. [, ]
Q4: Can you elaborate on the role of 2-iodothiophene in synthesizing substituted thiophenes?
A4: Researchers utilize 2-iodothiophene as a starting material to create a variety of substituted thiophenes through reactions like halogen-metal exchange and electrophilic aromatic substitution. For instance, reacting 2-iodothiophene with n-butyllithium generates 2-thienyllithium, a versatile intermediate for further functionalization. []
Q5: How is 2-iodothiophene employed in polymer chemistry?
A5: 2-Iodothiophene acts as a monomer in the synthesis of polythiophenes, a class of conducting polymers with applications in organic electronics and optoelectronics. [, ] The iodine atom can be further functionalized or removed after polymerization to fine-tune the polymer's properties.
Q6: What happens when 2-iodothiophene is exposed to UV light?
A6: Upon absorption of UV light, 2-iodothiophene undergoes C–I bond fission. [, , ] This process can occur via both direct and indirect dissociation pathways, leading to the formation of a thienyl radical and an iodine atom. [] The dynamics of this photodissociation have been studied using techniques like resonance Raman spectroscopy and time-resolved extreme ultraviolet (XUV) ionization. [, , ]
Q7: How has computational chemistry been used to study 2-iodothiophene?
A8: Computational methods like density functional theory (DFT) calculations have been utilized to investigate the electronic structure, excitation energies, and potential energy surfaces of 2-iodothiophene. [] These calculations provide valuable insights into the compound's photochemical behavior, including the mechanisms of photodissociation and the nature of the excited states involved. []
Q8: What is known about the stability of 2-iodothiophene?
A9: 2-Iodothiophene is sensitive to light and should be stored in the dark. [] It can undergo decomposition upon prolonged exposure to air and moisture. Proper handling and storage under inert atmosphere are recommended to maintain its purity and stability.
Q9: Are there studies on the material compatibility of 2-iodothiophene?
A10: Research has focused on incorporating 2-iodothiophene into various materials, including polymers. [, ] For example, its incorporation into methyl methacrylate copolymers for potential use in bone and dental cements has been explored. [] Understanding the compatibility and long-term stability of 2-iodothiophene within these materials is crucial for developing successful applications.
Descargo de responsabilidad e información sobre productos de investigación in vitro
Tenga en cuenta que todos los artículos e información de productos presentados en BenchChem están destinados únicamente con fines informativos. Los productos disponibles para la compra en BenchChem están diseñados específicamente para estudios in vitro, que se realizan fuera de organismos vivos. Los estudios in vitro, derivados del término latino "in vidrio", involucran experimentos realizados en entornos de laboratorio controlados utilizando células o tejidos. Es importante tener en cuenta que estos productos no se clasifican como medicamentos y no han recibido la aprobación de la FDA para la prevención, tratamiento o cura de ninguna condición médica, dolencia o enfermedad. Debemos enfatizar que cualquier forma de introducción corporal de estos productos en humanos o animales está estrictamente prohibida por ley. Es esencial adherirse a estas pautas para garantizar el cumplimiento de los estándares legales y éticos en la investigación y experimentación.