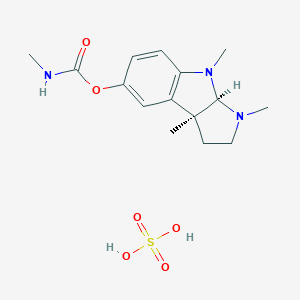
Hemisulfato de eserina
Descripción general
Descripción
Aplicaciones Científicas De Investigación
El sulfato de fisostigmina tiene una amplia gama de aplicaciones de investigación científica:
Química: Se utiliza como reactivo en diversas reacciones y estudios químicos.
Biología: El compuesto se utiliza para estudiar sistemas colinérgicos y neurotransmisión.
Mecanismo De Acción
El sulfato de fisostigmina actúa inhibiendo la acetilcolinesterasa, la enzima responsable de la descomposición de la acetilcolina. Al interferir con el metabolismo de la acetilcolina, el sulfato de fisostigmina estimula indirectamente tanto los receptores nicotínicos como los muscarínicos debido al aumento consecuente de la acetilcolina disponible en la sinapsis . Este mecanismo le permite revertir los efectos anticolinérgicos tanto centrales como periféricos .
Compuestos Similares:
Piridostigmina: Similar a la neostigmina, se utiliza para tratar la miastenia gravis, pero tiene una duración de acción más prolongada.
Unicidad: El sulfato de fisostigmina es único en su capacidad de atravesar la barrera hematoencefálica, lo que lo hace particularmente eficaz en el tratamiento de los efectos del sistema nervioso central de la toxicidad anticolinérgica . Esta propiedad lo distingue de otros inhibidores de la colinesterasa como la neostigmina y la piridostigmina .
Análisis Bioquímico
Biochemical Properties
Eserine Hemisulfate Salt plays a crucial role in biochemical reactions. It acts as an acetylcholinesterase inhibitor, forming a carbamylated enzyme complex with acetylcholinesterase that degrades slowly . This interaction with acetylcholinesterase is a key aspect of its biochemical activity .
Cellular Effects
The effects of Eserine Hemisulfate Salt on cells are profound. It influences cell function by interacting with acetylcholinesterase, an essential enzyme involved in neurotransmission . By inhibiting this enzyme, Eserine Hemisulfate Salt can affect cell signaling pathways, gene expression, and cellular metabolism .
Molecular Mechanism
Eserine Hemisulfate Salt exerts its effects at the molecular level primarily through its action as an acetylcholinesterase inhibitor . It forms a carbamylated enzyme complex with acetylcholinesterase, leading to slow degradation of the enzyme . This interaction can lead to changes in gene expression and influence various cellular processes .
Temporal Effects in Laboratory Settings
The effects of Eserine Hemisulfate Salt can change over time in laboratory settings. It is known to form a slowly degrading complex with acetylcholinesterase
Metabolic Pathways
Eserine Hemisulfate Salt is involved in the cholinergic system’s metabolic pathways through its action as an acetylcholinesterase inhibitor It can affect the levels of acetylcholine, a key neurotransmitter, in the body
Transport and Distribution
The transport and distribution of Eserine Hemisulfate Salt within cells and tissues are critical aspects of its function. As an acetylcholinesterase inhibitor, it is likely to be distributed wherever this enzyme is present . Specific details about its transporters or binding proteins are not currently available.
Subcellular Localization
The subcellular localization of Eserine Hemisulfate Salt can affect its activity and function. Given its role as an acetylcholinesterase inhibitor, it is likely to be found wherever this enzyme is localized within the cell
Métodos De Preparación
Rutas Sintéticas y Condiciones de Reacción: El sulfato de fisostigmina se puede sintetizar mediante varios métodos. Una ruta sintética común implica la condensación de 3-hidroxi-1-metilindol con cloruro de ácido N-metilcarbámico, seguida de ciclización y pasos de purificación posteriores .
Métodos de Producción Industrial: En entornos industriales, el sulfato de fisostigmina se produce típicamente mediante una serie de reacciones químicas que implican la extracción del compuesto activo del frijol de Calabar, seguida de purificación y conversión a la forma sulfato .
Análisis De Reacciones Químicas
Tipos de Reacciones: El sulfato de fisostigmina experimenta diversas reacciones químicas, que incluyen:
Oxidación: Esta reacción puede conducir a la formación de eserolina, un metabolito mayor.
Reducción: El sulfato de fisostigmina se puede reducir para formar diferentes derivados.
Sustitución: El compuesto puede sufrir reacciones de sustitución, particularmente en el grupo carbamato.
Reactivos y Condiciones Comunes:
Oxidación: Los agentes oxidantes comunes incluyen peróxido de hidrógeno y permanganato de potasio.
Reducción: A menudo se utilizan agentes reductores como el borohidruro de sodio.
Sustitución: Se pueden utilizar varios nucleófilos para reacciones de sustitución en condiciones controladas.
Productos Principales:
Oxidación: Eserolina
Reducción: Derivados reducidos de fisostigmina
Sustitución: Derivados de carbamato sustituidos
Comparación Con Compuestos Similares
Neostigmine: Another cholinesterase inhibitor, but it does not cross the blood-brain barrier as effectively as physostigmine sulfate.
Pyridostigmine: Similar to neostigmine, it is used to treat myasthenia gravis but has a longer duration of action.
Uniqueness: Physostigmine sulfate is unique in its ability to cross the blood-brain barrier, making it particularly effective in treating central nervous system effects of anticholinergic toxicity . This property distinguishes it from other cholinesterase inhibitors like neostigmine and pyridostigmine .
Propiedades
Número CAS |
64-47-1 |
---|---|
Fórmula molecular |
C15H23N3O6S |
Peso molecular |
373.4 g/mol |
Nombre IUPAC |
[(3aR,8bS)-3,4,8b-trimethyl-2,3a-dihydro-1H-pyrrolo[2,3-b]indol-7-yl] N-methylcarbamate;sulfuric acid |
InChI |
InChI=1S/C15H21N3O2.H2O4S/c1-15-7-8-17(3)13(15)18(4)12-6-5-10(9-11(12)15)20-14(19)16-2;1-5(2,3)4/h5-6,9,13H,7-8H2,1-4H3,(H,16,19);(H2,1,2,3,4)/t13-,15+;/m1./s1 |
Clave InChI |
LLIODTIILSCNOH-PBCQUBLHSA-N |
SMILES |
CC12CCN(C1N(C3=C2C=C(C=C3)OC(=O)NC)C)C.CC12CCN(C1N(C3=C2C=C(C=C3)OC(=O)NC)C)C.OS(=O)(=O)O |
SMILES isomérico |
C[C@@]12CCN([C@@H]1N(C3=C2C=C(C=C3)OC(=O)NC)C)C.OS(=O)(=O)O |
SMILES canónico |
CC12CCN(C1N(C3=C2C=C(C=C3)OC(=O)NC)C)C.OS(=O)(=O)O |
Key on ui other cas no. |
64-47-1 |
Pictogramas |
Acute Toxic |
Sinónimos |
(3aS-cis)-1,2,3,3a,8,8a-Hexahydro-1,3a,8-trimethyl-pyrrolo[2,3-b]indol-5-ol Methylcarbamate (Ester) Sulfate (Salt) |
Origen del producto |
United States |
Retrosynthesis Analysis
AI-Powered Synthesis Planning: Our tool employs the Template_relevance Pistachio, Template_relevance Bkms_metabolic, Template_relevance Pistachio_ringbreaker, Template_relevance Reaxys, Template_relevance Reaxys_biocatalysis model, leveraging a vast database of chemical reactions to predict feasible synthetic routes.
One-Step Synthesis Focus: Specifically designed for one-step synthesis, it provides concise and direct routes for your target compounds, streamlining the synthesis process.
Accurate Predictions: Utilizing the extensive PISTACHIO, BKMS_METABOLIC, PISTACHIO_RINGBREAKER, REAXYS, REAXYS_BIOCATALYSIS database, our tool offers high-accuracy predictions, reflecting the latest in chemical research and data.
Strategy Settings
Precursor scoring | Relevance Heuristic |
---|---|
Min. plausibility | 0.01 |
Model | Template_relevance |
Template Set | Pistachio/Bkms_metabolic/Pistachio_ringbreaker/Reaxys/Reaxys_biocatalysis |
Top-N result to add to graph | 6 |
Feasible Synthetic Routes
Q1: How does eserine sulfate exert its effects?
A1: Eserine sulfate functions as a reversible cholinesterase inhibitor. [, , ] It interacts with the active site of the enzyme acetylcholinesterase, preventing the breakdown of acetylcholine. [, , , , ] This inhibition leads to an accumulation of acetylcholine at cholinergic synapses, resulting in prolonged and enhanced cholinergic neurotransmission. [, , , , , , , ]
Q2: What are the downstream effects of this interaction?
A2: The accumulation of acetylcholine due to eserine sulfate inhibition can have various effects on different organ systems, including:
- Muscular System: Increased muscle contractions and potential for muscle paralysis at high concentrations. [, , , ]
- Nervous System: Enhanced cognitive function, increased alertness, and potential for seizures at high concentrations. [, , , , , ]
- Ocular System: Pupil constriction (miosis), increased accommodation, and potential for blurred vision. [, , ]
- Respiratory System: Bronchoconstriction and increased mucus secretion. []
- Gastrointestinal System: Increased intestinal motility and secretions. []
Q3: What is the molecular formula and weight of eserine sulfate?
A3: The molecular formula of eserine sulfate is (C15H21N3O2)2.H2SO4. Its molecular weight is 648.8 g/mol. [, , ]
Q4: Is there any spectroscopic data available for eserine sulfate?
A4: Yes, research has employed various spectroscopic techniques to characterize eserine sulfate, including infrared spectroscopy. [, ] These analyses provide valuable information regarding the compound's functional groups and structural characteristics.
Q5: How does eserine sulfate perform under different conditions?
A5: Eserine sulfate exhibits stability at specific pH ranges and temperatures. [, , , ] Research has explored its formulation into various delivery systems, such as micronized emulsions, to enhance its stability and bioavailability. [, ]
Q6: What are the primary applications of eserine sulfate?
A6: Eserine sulfate finds applications in various research areas, including:
- Neurological Research: Investigating cholinergic pathways and their role in cognitive function, memory, and sleep. [, , , , ]
- Ophthalmological Research: Inducing miosis for diagnostic and therapeutic purposes, such as in the treatment of glaucoma. [, ]
- Pharmacological Research: Evaluating its potential as an antidote for organophosphate poisoning. []
Q7: What animal models are used to study eserine sulfate?
A7: Research on eserine sulfate commonly employs various animal models, including rats, mice, rabbits, guinea pigs, dogs, and frogs. [, , , , , , , , ] These models provide valuable insights into the compound's effects on different physiological systems.
Q8: What are the known toxicological properties of eserine sulfate?
A8: Eserine sulfate can exhibit toxicity at high doses, with potential adverse effects on the muscular, nervous, respiratory, and cardiovascular systems. [, , ] Research emphasizes the importance of careful dose selection and administration to mitigate potential risks.
Q9: What are the future directions for research on eserine sulfate?
A9: Ongoing research on eserine sulfate focuses on:
- Targeted Drug Delivery: Developing novel delivery systems to enhance its efficacy and minimize potential side effects. []
- Biomarker Discovery: Identifying biomarkers to predict treatment response and monitor potential adverse effects. []
- Understanding Resistance Mechanisms: Investigating potential resistance mechanisms to cholinesterase inhibitors and exploring strategies to overcome them. [, ]
Descargo de responsabilidad e información sobre productos de investigación in vitro
Tenga en cuenta que todos los artículos e información de productos presentados en BenchChem están destinados únicamente con fines informativos. Los productos disponibles para la compra en BenchChem están diseñados específicamente para estudios in vitro, que se realizan fuera de organismos vivos. Los estudios in vitro, derivados del término latino "in vidrio", involucran experimentos realizados en entornos de laboratorio controlados utilizando células o tejidos. Es importante tener en cuenta que estos productos no se clasifican como medicamentos y no han recibido la aprobación de la FDA para la prevención, tratamiento o cura de ninguna condición médica, dolencia o enfermedad. Debemos enfatizar que cualquier forma de introducción corporal de estos productos en humanos o animales está estrictamente prohibida por ley. Es esencial adherirse a estas pautas para garantizar el cumplimiento de los estándares legales y éticos en la investigación y experimentación.