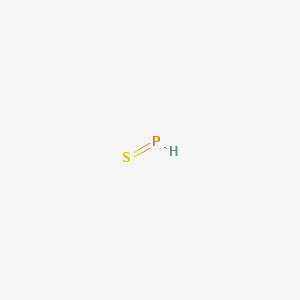
Phosphorus sulfide
Descripción general
Descripción
Phosphorus sulfides comprise a family of inorganic compounds containing only phosphorus and sulfur. These compounds have the formula P4Sn with n ≤ 10. Two are of commercial significance, phosphorus pentasulfide (P4S10), which is made on a kiloton scale for the production of other organosulfur compounds, and phosphorus sesquisulfide (P4S3), used in the production of "strike anywhere matches" .
Synthesis Analysis
The main method for preparing these compounds is thermolysis of mixtures of phosphorus and sulfur. The product distributions can be analyzed by 31 P-NMR spectroscopy. More selective syntheses entail desulfurization, e.g., using triphenylphosphine and, complementarily, sulfidation using triphenylarsine sulfide .
Molecular Structure Analysis
All known molecular phosphorus sulfides contain a tetrahedral array of four phosphorus atoms . Its tetrahedral molecular structure is similar to that of adamantane and almost identical to the structure of phosphorus pentoxide .
Chemical Reactions Analysis
Phosphorus-centered radicals are closely related to human life and health, and are widely used in biology, chemistry, functional materials, and other fields; they play a key role in the processes of bond cleavage and formation .
Physical And Chemical Properties Analysis
Phosphorus trisulfide, free from yellow and white phosphorus appears as a grayish-yellow solid . The phosphorus sulfide is easily ignited, the potassium chlorate decomposes to give oxygen, which in turn causes the phosphorus sulfide to burn more vigorously .
Aplicaciones Científicas De Investigación
Synthesis of Monodispersed Nanoparticles
Utilizing high-temperature thermo-decomposition methods, phosphorus sulfide is used in the synthesis of highly monodispersed, (super)paramagnetic nanoparticles . These nanoparticles have significant potential in various fields, including medical imaging and data storage.
Thermochemical Studies
Phosphorus sulfide compounds, particularly their cage-like structures, pose an extreme challenge for high-level ab initio methods in thermochemical studies . These studies are crucial for understanding the stability and reactivity of these compounds.
Enhancing Phosphorus Absorption in Agriculture
Research indicates that phosphorus sulfide could play a role in enhancing phosphorus absorption and transportation in rice, which is vital for plant growth and crop yield .
Mecanismo De Acción
Mode of Action
It is known that many sulfonamides act as competitive inhibitors of bacterial enzyme dihydropteroate synthetase . This enzyme normally uses para-aminobenzoic acid (PABA) for synthesizing the necessary folic acid .
Biochemical Pathways
It is known that sulfonamides and related compounds can affect the synthesis of folic acid, which is essential for the production of dna in bacteria . Phosphorylation and sulfation share a common biosynthetic pathway . The sulfate donor 30-phosphoadenosine-50-phosphosulfate is formed from adenosine triphosphate (ATP) and adenosine phospho-sulfate .
Action Environment
It is known that environmental factors can significantly impact the action of many chemical compounds .
Safety and Hazards
Direcciones Futuras
Sulfide-based solid-state electrolytes (SSEs) have attracted much attention owing to their high ionic conductivity and feasible mechanical features. The environmental stability of sulfide-based SSEs is one of the critical aspects due to the possible decomposition, and ionic conductivity change will affect the fabrication and electrochemical performance of the batteries . Unsustainable phosphorus use is pushing food security further from reach, leaving a legacy of polluted freshwaters, many now beyond ecological restoration .
Propiedades
IUPAC Name |
sulfanylidenephosphane | |
---|---|---|
Source | PubChem | |
URL | https://pubchem.ncbi.nlm.nih.gov | |
Description | Data deposited in or computed by PubChem | |
InChI |
InChI=1S/HPS/c1-2/h1H | |
Source | PubChem | |
URL | https://pubchem.ncbi.nlm.nih.gov | |
Description | Data deposited in or computed by PubChem | |
InChI Key |
OKQKDCXVLPGWPO-UHFFFAOYSA-N | |
Source | PubChem | |
URL | https://pubchem.ncbi.nlm.nih.gov | |
Description | Data deposited in or computed by PubChem | |
Canonical SMILES |
P=S | |
Source | PubChem | |
URL | https://pubchem.ncbi.nlm.nih.gov | |
Description | Data deposited in or computed by PubChem | |
Molecular Formula |
HPS | |
Source | PubChem | |
URL | https://pubchem.ncbi.nlm.nih.gov | |
Description | Data deposited in or computed by PubChem | |
Molecular Weight |
64.05 g/mol | |
Source | PubChem | |
URL | https://pubchem.ncbi.nlm.nih.gov | |
Description | Data deposited in or computed by PubChem | |
Product Name |
Phosphine sulfide |
Synthesis routes and methods I
Procedure details
Synthesis routes and methods II
Procedure details
Q & A
Q1: What are some common molecular formulas of phosphorus sulfides?
A1: Research has identified a variety of phosphorus sulfides, with common examples including tetraphosphorus trisulfide (P4S3), tetraphosphorus pentasulfide (P4S5) which exists in α and β forms, tetraphosphorus heptasulfide (P4S7), and tetraphosphorus decasulfide (P4S10). [, , , , ]
Q2: How can phosphorus sulfides be characterized?
A2: Various spectroscopic techniques are employed for characterization, with 31P NMR spectroscopy being particularly valuable due to the 100% natural abundance of the 31P isotope. This technique allows for the identification of different phosphorus environments within the molecules. Additionally, Raman spectroscopy provides complementary information on vibrational modes, aiding in structural elucidation. [, , , ]
Q3: Do phosphorus sulfide structures differ in the solid and liquid states?
A3: Yes, some phosphorus sulfides exhibit polymorphism, meaning they can exist in different crystal structures in the solid state. For instance, both β-P4S5 and β-P4S6 have been found to exist in two distinct polymorphs. These structural variations can influence their physical and chemical properties. High-speed 31P MAS-NMR spectroscopy is a valuable tool for characterizing these solid-state structures. []
Q4: How are phosphorus sulfides synthesized?
A4: One common method involves the direct reaction of elemental phosphorus (P4) with sulfur (S8) at elevated temperatures. This reaction yields a mixture of phosphorus sulfides, with the product distribution influenced by factors such as temperature and reaction time. [, , ]
Q5: How do phosphorus sulfides react with nucleophiles?
A6: Phosphorus sulfides are susceptible to nucleophilic attack. For example, reactions with fluorides like sodium fluoride (NaF) or potassium fluoride (KF) in solvents like acetonitrile lead to the formation of sulfane-α,ω-diyl-bis(fluorodithiophosphates). The chain length of the sulfane derivatives formed is dependent on the initial sulfur-to-phosphorus ratio in the starting phosphorus sulfide. []
Q6: What is the role of phosphorus sulfides in organic synthesis?
A7: Phosphorus sulfides, particularly tetraphosphorus decasulfide (P4S10), find applications in organic synthesis for thionation reactions. They can convert carbonyl groups (C=O) into thiocarbonyl groups (C=S). For instance, P4S10 can be used to synthesize thioamides from amides. [, , ]
Q7: Can phosphorus sulfides be used as precursors for thin film deposition?
A9: Yes, research has demonstrated the use of plasma-enhanced chemical vapor deposition (PECVD) for depositing amorphous silicon sulfide (SiSx:H) thin films using hydrogen sulfide (H2S) and silane (SiH4) as precursors. This method offers control over the film's composition and structure by adjusting deposition parameters. Preliminary studies also suggest the feasibility of using PECVD for phosphorus sulfide thin film deposition. []
Q8: Are there alternative materials being explored for similar applications?
A10: Yes, in the field of two-dimensional (2D) materials, silver indium phosphorus sulfide (AgInP2S6) is emerging as a promising candidate for nonlinear optical applications. This 2D material exhibits second-harmonic generation (SHG), a nonlinear optical effect with potential applications in areas like frequency conversion and optical sensing. []
Q9: Are there any safety considerations when working with phosphorus sulfides?
A11: Yes, phosphorus sulfides, particularly the lower sulfides like P4S3, are flammable and can ignite spontaneously in air. It's crucial to handle these compounds with care, employing appropriate safety measures such as working in well-ventilated areas and using protective equipment. []
Q10: What are the environmental concerns associated with phosphorus sulfides?
A12: While specific ecotoxicological data might be limited for some phosphorus sulfides, it's important to consider their potential impact on the environment. Responsible waste management practices are essential to prevent contamination of soil and water resources. [, ]
Descargo de responsabilidad e información sobre productos de investigación in vitro
Tenga en cuenta que todos los artículos e información de productos presentados en BenchChem están destinados únicamente con fines informativos. Los productos disponibles para la compra en BenchChem están diseñados específicamente para estudios in vitro, que se realizan fuera de organismos vivos. Los estudios in vitro, derivados del término latino "in vidrio", involucran experimentos realizados en entornos de laboratorio controlados utilizando células o tejidos. Es importante tener en cuenta que estos productos no se clasifican como medicamentos y no han recibido la aprobación de la FDA para la prevención, tratamiento o cura de ninguna condición médica, dolencia o enfermedad. Debemos enfatizar que cualquier forma de introducción corporal de estos productos en humanos o animales está estrictamente prohibida por ley. Es esencial adherirse a estas pautas para garantizar el cumplimiento de los estándares legales y éticos en la investigación y experimentación.