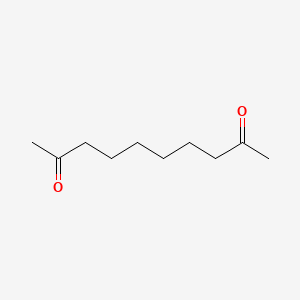
Decano-2,9-diona
Descripción general
Descripción
Decane-2,9-dione is an organic compound with the molecular formula C10H18O2 It is a diketone, meaning it contains two ketone functional groups located at the 2nd and 9th positions of the decane chain
Aplicaciones Científicas De Investigación
Decane-2,9-dione has several applications in scientific research:
Chemistry: It is used as a building block in organic synthesis, particularly in the preparation of complex molecules.
Biology: The compound is studied for its potential biological activity and interactions with enzymes.
Medicine: Research is ongoing to explore its potential therapeutic applications, including its use as a precursor for drug synthesis.
Industry: Decane-2,9-dione is used in the production of polymers and other industrial chemicals.
Mecanismo De Acción
Target of Action
Decane-2,9-dione has been identified as a promising inhibitor of the Receptor-interacting protein kinase 1 (RIPK1) . RIPK1 is a key component of the necroptosis signaling pathway, a type of programmed cell death . This pathway is involved in various pathophysiological disorders, including inflammatory, neurodegenerative, infectious, and malignant diseases .
Mode of Action
The compound interacts with RIPK1 by inserting its benzyl groups into the bottom of a deep hydrophobic pocket in RIPK1, forming T-shaped π–π interactions with His136 . This interaction inhibits the function of RIPK1, thereby disrupting the necroptosis signaling pathway .
Biochemical Pathways
The necroptosis signaling pathway is initiated by signals from death receptors in the tumor necrosis factor (TNF) superfamily, Toll-like receptors (TLRs), interferon receptors (IFNAR), or Z-DNA binding protein 1 (ZBP1) . Downstream of death receptor signaling, serine/threonine kinases, RIPK1 and RIPK3, as well as mixed lineage kinase domain-like (MLKL), are key components in the necroptosis signaling pathway . By inhibiting RIPK1, Decane-2,9-dione disrupts this pathway, potentially preventing the cell death and inflammation associated with necroptosis .
Result of Action
The inhibition of RIPK1 by Decane-2,9-dione disrupts the necroptosis signaling pathway, potentially preventing the cell death and inflammation associated with necroptosis . This could have therapeutic implications for various diseases involving necroptosis, including inflammatory, neurodegenerative, infectious, and malignant diseases .
Métodos De Preparación
Synthetic Routes and Reaction Conditions
Decane-2,9-dione can be synthesized through several methods. One common approach involves the oxidation of decane-2,9-diol using an oxidizing agent such as pyridinium chlorochromate (PCC) or Jones reagent. The reaction typically takes place under mild conditions, with the diol being dissolved in an appropriate solvent like dichloromethane.
Industrial Production Methods
In an industrial setting, decane-2,9-dione can be produced through the catalytic dehydrogenation of decane-2,9-diol. This process involves passing the diol over a metal catalyst, such as copper or palladium, at elevated temperatures. The resulting diketone is then purified through distillation or recrystallization.
Análisis De Reacciones Químicas
Types of Reactions
Decane-2,9-dione undergoes various chemical reactions, including:
Oxidation: The diketone can be further oxidized to produce carboxylic acids.
Reduction: It can be reduced to form decane-2,9-diol using reducing agents like sodium borohydride or lithium aluminum hydride.
Substitution: The ketone groups can participate in nucleophilic substitution reactions, forming various derivatives.
Common Reagents and Conditions
Oxidation: Common oxidizing agents include potassium permanganate and chromium trioxide.
Reduction: Reducing agents such as sodium borohydride and lithium aluminum hydride are frequently used.
Substitution: Nucleophiles like amines and alcohols can react with the ketone groups under acidic or basic conditions.
Major Products Formed
Oxidation: Carboxylic acids.
Reduction: Decane-2,9-diol.
Substitution: Various substituted derivatives depending on the nucleophile used.
Comparación Con Compuestos Similares
Similar Compounds
Decane-2,9-diol: The reduced form of decane-2,9-dione, containing hydroxyl groups instead of ketone groups.
Decane-2,9-dicarboxylic acid: The oxidized form, containing carboxylic acid groups.
6,10-Dioxaspiro[4.5]decane-7,9-dione: A spirocyclic compound with similar structural features.
Uniqueness
Decane-2,9-dione is unique due to its specific positioning of the ketone groups, which imparts distinct chemical properties and reactivity. This makes it a valuable compound for various synthetic and research applications.
Actividad Biológica
Decane-2,9-dione (C10H18O2) is a diketone compound that has garnered attention in recent years for its potential biological activities. This article explores its biological activity, mechanisms of action, and relevant research findings, including data tables and case studies.
Overview of Decane-2,9-dione
Decane-2,9-dione is characterized by two ketone functional groups located at the 2nd and 9th positions of the decane chain. This unique structure contributes to its distinctive chemical properties and reactivity. The compound has been studied for its interactions with enzymes and potential therapeutic applications.
Target of Action : Decane-2,9-dione has been identified as an inhibitor of the Receptor-interacting protein kinase 1 (RIPK1), a key player in necroptosis—a form of programmed cell death associated with inflammation and various diseases.
Mode of Action : The compound interacts with RIPK1 by inserting its benzyl groups into a hydrophobic pocket within the enzyme, forming T-shaped π–π interactions with the amino acid His136. This interaction disrupts the necroptosis signaling pathway, potentially preventing cell death and associated inflammation.
Biological Activities
Research indicates that decane-2,9-dione exhibits several biological activities:
- Anti-inflammatory Effects : By inhibiting RIPK1, decane-2,9-dione may reduce inflammation linked to necroptosis.
- Antimicrobial Properties : Some studies suggest that derivatives of decane-2,9-dione show significant antimicrobial activity against various pathogens.
- Potential Anticancer Activity : The inhibition of RIPK1 may also have implications in cancer therapy by preventing necroptosis in tumor cells.
Case Studies
- Inhibition of RIPK1 : A study demonstrated that decane-2,9-dione effectively inhibited RIPK1 activity in vitro, leading to reduced necroptotic cell death in human cell lines exposed to inflammatory stimuli.
- Antimicrobial Activity : In another investigation, derivatives of decane-2,9-dione were tested against bacterial strains such as Staphylococcus aureus and Escherichia coli, showing promising results in inhibiting growth.
Data Table: Biological Activities of Decane-2,9-dione
Biological Activity | Effectiveness | Reference |
---|---|---|
RIPK1 Inhibition | High | |
Antimicrobial Activity | Moderate | |
Anti-inflammatory Effects | High |
Chemical Reactions
Decane-2,9-dione can undergo various chemical reactions that may influence its biological activity:
- Oxidation : Can be oxidized to produce carboxylic acids.
- Reduction : Can be reduced to form decane-2,9-diol.
- Substitution Reactions : The diketone can participate in nucleophilic substitutions, forming various derivatives that may enhance or alter its biological properties.
Propiedades
IUPAC Name |
decane-2,9-dione | |
---|---|---|
Source | PubChem | |
URL | https://pubchem.ncbi.nlm.nih.gov | |
Description | Data deposited in or computed by PubChem | |
InChI |
InChI=1S/C10H18O2/c1-9(11)7-5-3-4-6-8-10(2)12/h3-8H2,1-2H3 | |
Source | PubChem | |
URL | https://pubchem.ncbi.nlm.nih.gov | |
Description | Data deposited in or computed by PubChem | |
InChI Key |
YUMJAVUEKNJIQY-UHFFFAOYSA-N | |
Source | PubChem | |
URL | https://pubchem.ncbi.nlm.nih.gov | |
Description | Data deposited in or computed by PubChem | |
Canonical SMILES |
CC(=O)CCCCCCC(=O)C | |
Source | PubChem | |
URL | https://pubchem.ncbi.nlm.nih.gov | |
Description | Data deposited in or computed by PubChem | |
Molecular Formula |
C10H18O2 | |
Source | PubChem | |
URL | https://pubchem.ncbi.nlm.nih.gov | |
Description | Data deposited in or computed by PubChem | |
DSSTOX Substance ID |
DTXSID20336939 | |
Record name | decane-2,9-dione | |
Source | EPA DSSTox | |
URL | https://comptox.epa.gov/dashboard/DTXSID20336939 | |
Description | DSSTox provides a high quality public chemistry resource for supporting improved predictive toxicology. | |
Molecular Weight |
170.25 g/mol | |
Source | PubChem | |
URL | https://pubchem.ncbi.nlm.nih.gov | |
Description | Data deposited in or computed by PubChem | |
CAS No. |
16538-91-3 | |
Record name | decane-2,9-dione | |
Source | EPA DSSTox | |
URL | https://comptox.epa.gov/dashboard/DTXSID20336939 | |
Description | DSSTox provides a high quality public chemistry resource for supporting improved predictive toxicology. | |
Record name | Decane-2,9-dione | |
Source | European Chemicals Agency (ECHA) | |
URL | https://echa.europa.eu/information-on-chemicals | |
Description | The European Chemicals Agency (ECHA) is an agency of the European Union which is the driving force among regulatory authorities in implementing the EU's groundbreaking chemicals legislation for the benefit of human health and the environment as well as for innovation and competitiveness. | |
Explanation | Use of the information, documents and data from the ECHA website is subject to the terms and conditions of this Legal Notice, and subject to other binding limitations provided for under applicable law, the information, documents and data made available on the ECHA website may be reproduced, distributed and/or used, totally or in part, for non-commercial purposes provided that ECHA is acknowledged as the source: "Source: European Chemicals Agency, http://echa.europa.eu/". Such acknowledgement must be included in each copy of the material. ECHA permits and encourages organisations and individuals to create links to the ECHA website under the following cumulative conditions: Links can only be made to webpages that provide a link to the Legal Notice page. | |
Retrosynthesis Analysis
AI-Powered Synthesis Planning: Our tool employs the Template_relevance Pistachio, Template_relevance Bkms_metabolic, Template_relevance Pistachio_ringbreaker, Template_relevance Reaxys, Template_relevance Reaxys_biocatalysis model, leveraging a vast database of chemical reactions to predict feasible synthetic routes.
One-Step Synthesis Focus: Specifically designed for one-step synthesis, it provides concise and direct routes for your target compounds, streamlining the synthesis process.
Accurate Predictions: Utilizing the extensive PISTACHIO, BKMS_METABOLIC, PISTACHIO_RINGBREAKER, REAXYS, REAXYS_BIOCATALYSIS database, our tool offers high-accuracy predictions, reflecting the latest in chemical research and data.
Strategy Settings
Precursor scoring | Relevance Heuristic |
---|---|
Min. plausibility | 0.01 |
Model | Template_relevance |
Template Set | Pistachio/Bkms_metabolic/Pistachio_ringbreaker/Reaxys/Reaxys_biocatalysis |
Top-N result to add to graph | 6 |
Feasible Synthetic Routes
Q1: What is the significance of converting Decane-2,9-dione to (Z,Z)-3,8-bis[1-(difluoroboryloxy)ethylidene]decane-2,9-dione as described in the research?
A1: The conversion of Decane-2,9-dione to (Z,Z)-3,8-bis[1-(difluoroboryloxy)ethylidene]decane-2,9-dione, achieved through a multi-step synthesis involving enol acetate formation and reaction with a boron trifluoride-acetic acid complex, allows for the study of its crystal structure. [] This provides valuable insights into the molecular geometry and arrangement of the compound, contributing to a deeper understanding of its chemical properties and potential applications. [] You can find the details of this synthesis and the resulting crystal structure analysis in the paper "Crystal structure of (Z,Z)-3,8-bis[1-(difluoroboryloxy)ethylidene]decane- 2,9-dione, C10H14O2(C2H3OBF2)2". []
Q2: How is Decane-2,9-dione typically synthesized, and what is its relevance in organic chemistry?
A2: Decane-2,9-dione can be synthesized with a good yield (83%) by reacting octanedioic chloride with the magnesium enolate of diethyl malonate. [] This diketone serves as a valuable precursor in organic synthesis. For instance, it can be used to create bicyclo[4,3,1]decane-2,9-dione, a key intermediate in studying "anti-Bredt" alkenes, which are molecules that violate Bredt's Rule. [] This rule predicts the stability and likelihood of forming bridgehead double bonds in bridged ring systems. Therefore, Decane-2,9-dione plays a crucial role in exploring the boundaries and exceptions to this fundamental principle in organic chemistry.
Q3: Can you describe the molecular structure of (Z,Z)-3,8-bis[1-(difluoroboryloxy)ethylidene]decane-2,9-dione based on the provided data?
A3: (Z,Z)-3,8-bis[1-(difluoroboryloxy)ethylidene]decane-2,9-dione crystallizes in the monoclinic system with the space group P\2\/n. [] The unit cell dimensions are a = 7.691(1) Å, b = 11.482(2) Å, c = 9.997(1) Å, and β = 106.22(1)°. [] The crystal structure reveals the specific arrangement of atoms within the molecule, including bond lengths, angles, and intermolecular interactions. This information is crucial for understanding the compound's physical properties, such as melting point and solubility.
Descargo de responsabilidad e información sobre productos de investigación in vitro
Tenga en cuenta que todos los artículos e información de productos presentados en BenchChem están destinados únicamente con fines informativos. Los productos disponibles para la compra en BenchChem están diseñados específicamente para estudios in vitro, que se realizan fuera de organismos vivos. Los estudios in vitro, derivados del término latino "in vidrio", involucran experimentos realizados en entornos de laboratorio controlados utilizando células o tejidos. Es importante tener en cuenta que estos productos no se clasifican como medicamentos y no han recibido la aprobación de la FDA para la prevención, tratamiento o cura de ninguna condición médica, dolencia o enfermedad. Debemos enfatizar que cualquier forma de introducción corporal de estos productos en humanos o animales está estrictamente prohibida por ley. Es esencial adherirse a estas pautas para garantizar el cumplimiento de los estándares legales y éticos en la investigación y experimentación.