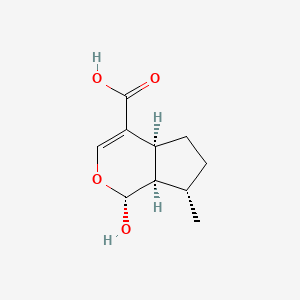
7-Deoxyloganetic acid
- Haga clic en CONSULTA RÁPIDA para recibir una cotización de nuestro equipo de expertos.
- Con productos de calidad a un precio COMPETITIVO, puede centrarse más en su investigación.
Descripción general
Descripción
7-deoxyloganetic acid is an iridoid monoterpenoid that is 1,4a,5,6,7,7a-hexahydrocyclopenta[c]pyran-4-carboxylic acid substituted at positions 1 and 7 by hydroxy and methyl groups respectively; the aglycone of 7-deoxyloganic acid. It has a role as a plant metabolite. It is an iridoid monoterpenoid, a cyclopentapyran and an alpha,beta-unsaturated monocarboxylic acid. It is a conjugate acid of a 7-deoxyloganetate.
Aplicaciones Científicas De Investigación
Biosynthesis of Monoterpenoid Indole Alkaloids
7-Deoxyloganetic acid plays a crucial role in the biosynthesis of MIAs, which are important for their medicinal properties. The compound is synthesized through a series of enzymatic reactions involving specific enzymes such as this compound synthase (7DLS) and this compound glucosyltransferase (7DLGT).
Key Studies:
- A study identified the enzyme this compound synthase (CYP76A26) in Catharanthus roseus, which catalyzes the three-step oxidation necessary for producing this compound from iridodial-nepetalactol. This discovery is pivotal for enhancing MIA production in various biological systems .
- Another research highlighted the functional characterization of two cytochrome P450 enzymes from Camptotheca acuminata, demonstrating their role in the oxidation process leading to this compound synthesis .
Medicinal Applications
The MIAs derived from this compound are known for their anticancer properties. Compounds like vinblastine and vincristine, which are derived from MIAs, are widely used in cancer therapy.
Case Studies:
- The medicinal relevance of Catharanthus roseus is attributed to its alkaloids, which disrupt cell division by binding to microtubules, making them effective for treating various cancers .
- Research has also explored repositioning cannabinoids and terpenes, including this compound, as potential candidates for targeted cancer therapies, showcasing its versatility in medicinal applications .
Genetic Engineering and Biotechnology
The manipulation of genes involved in the biosynthesis of this compound presents opportunities for biotechnological advancements.
Research Findings:
- Virus-induced gene silencing techniques have been employed to study the effects of silencing genes associated with this compound synthesis. This approach has shown significant reductions in secologanin levels while increasing this compound concentrations .
- Functional assays conducted in Saccharomyces cerevisiae have confirmed the enzymatic activities related to this compound synthesis, paving the way for synthetic biology applications .
Enzymatic Pathways and Mechanisms
Understanding the enzymatic pathways that lead to the formation of this compound is essential for optimizing its production.
Detailed Insights:
- The pathway involves several steps where specific enzymes catalyze reactions leading to the conversion of precursors into this compound and subsequently into other valuable metabolites .
- The identification of glucosyltransferases that act on this compound has been crucial in elucidating its role in further metabolic pathways, contributing to enhanced production strategies for MIAs .
Análisis De Reacciones Químicas
Enzymatic Glucosylation to 7-Deoxyloganic Acid
7-Deoxyloganetic acid undergoes glucosylation via UDP-glucose-dependent glucosyltransferases (UGTs) to form 7-deoxyloganic acid. This reaction is a pivotal step in the secologanin biosynthetic pathway:
-
UGT8 (Catharanthus roseus) : Exhibits high catalytic efficiency for this compound, with a K<sub>m</sub> of 0.088 ± 0.022 mM and k<sub>cat</sub> of 0.130 ± 0.015 s<sup>−1</sup>, yielding a catalytic efficiency (k<sub>cat</sub>/K<sub>m</sub>) of 1,523.3 ± 222.7 M<sup>−1</sup>s<sup>−1</sup> .
-
UGT709C2 (C. roseus) : Catalyzes the same reaction with a K<sub>m<sup>app</sup></sub> of 9.8 µM and k<sub>cat</sub> of 1.25 s<sup>−1</sup>, demonstrating substrate specificity for this compound .
Table 1: Kinetic Parameters of Glucosyltransferases
Enzyme | Substrate | K<sub>m</sub> (mM) | k<sub>cat</sub> (s<sup>−1</sup>) | k<sub>cat</sub>/K<sub>m</sub> (M<sup>−1</sup>s<sup>−1</sup>) |
---|---|---|---|---|
UGT8 | This compound | 0.088 ± 0.022 | 0.130 ± 0.015 | 1,523.3 ± 222.7 |
UGT709C2 | This compound | 0.0098 | 1.25 | 127,551 |
Role in Biosynthetic Pathways
This compound is a precursor in the seco-iridoid pathway , leading to loganic acid, loganin, and secologanin. Key steps include:
-
Hydroxylation : 7-Deoxyloganic acid (the glucosylated product) is hydroxylated by CYP72A224 to form loganic acid, a precursor for secologanin .
-
Gene Silencing Effects : Silencing CrDL7H (7-deoxyloganic acid hydroxylase) in C. roseus reduces secologanin levels by >70% and increases 7-deoxyloganic acid accumulation to >4 mg/g fresh leaf weight .
Substrate Specificity and Competing Reactions
-
UGT6 and UGT7 : These glucosyltransferases show negligible activity toward this compound, with catalytic efficiencies (k<sub>cat</sub>/K<sub>m</sub>) of 179.9 ± 54.5 M<sup>−1</sup>s<sup>−1</sup> (UGT6) and 2.35 ± 0.58 M<sup>−1</sup>s<sup>−1</sup> (UGT7) for related substrates .
-
Iridoid Oxidase (IO) : Produces this compound from nepetalactol or iridodial, though this step precedes its glucosylation .
Structural and Functional Insights
-
Molecular Structure : this compound (C<sub>10</sub>H<sub>14</sub>O<sub>4</sub>) features a cyclopentane-pyran core with carboxylic acid and hydroxyl groups, critical for enzyme recognition .
-
Mechanistic Studies : Computational models suggest that UGT8’s active site accommodates this compound via hydrogen bonding with UDP-glucose, facilitating efficient glucosyl transfer .
Q & A
Basic Research Questions
Q. What are the key enzymatic steps in the biosynthesis of 7-deoxyloganetic acid in Catharanthus roseus?
The biosynthesis involves two critical enzymes: iridoid oxidase (IO/CYP76A2) and alcohol dehydrogenase (ADH). IO catalyzes the oxidation of cis-trans-nepetalactol to this compound, while ADH facilitates redox adjustments. Functional validation of these enzymes requires heterologous expression in yeast or E. coli, followed by enzyme assays using HPLC or LC-MS to quantify substrate conversion . Notably, IO from Catharanthus roseus (CrolO) is essential, and variants with ≥70% sequence identity retain activity when co-expressed with ADH .
Q. How can researchers optimize heterologous production of this compound in engineered yeast strains?
Key steps include:
- Co-expressing CrolO and ADH with codon optimization for yeast compatibility.
- Supplementing media with precursors like 8-oxogeranial and tryptamine to bypass endogenous metabolic limitations.
- Monitoring titers via LC-MS, with successful strains achieving ≥10 μM this compound .
- Iterative strain engineering (e.g., promoter tuning, redox balancing) to enhance flux toward the target compound .
Q. What analytical methods are recommended for quantifying this compound in plant extracts or microbial cultures?
Use LC-MS/MS with multiple reaction monitoring (MRM) for high specificity. For structural confirmation, employ NMR (e.g., 1H, 13C, and 2D-COSY) to resolve the cyclopenta[c]pyran core and carboxylate moiety. Reference standards should be isolated from Catharanthus roseus or synthesized enzymatically .
Advanced Research Questions
Q. How can conflicting data on the order of glycosylation vs. methylation in this compound metabolism be resolved?
Studies in Gardenia jasminoides suggest glycosylation precedes methylation, while Catharanthus roseus data indicate methylation occurs first. To address this:
- Perform in vitro enzyme assays with purified this compound glucosyltransferase (7DLGT) and loganic acid methyltransferase (LAMT) using isotopically labeled substrates.
- Use tissue-specific RNA-seq to correlate spatiotemporal expression of 7DLGT and LAMT with metabolite profiles .
- Apply kinetic modeling to determine preferential substrate binding (e.g., this compound vs. 7-deoxyloganetin) .
Q. What experimental strategies can elucidate the role of this compound in cross-species variability of terpenoid indole alkaloid (TIA) biosynthesis?
- Compare transcriptomes and metabolomes of high-TIA species (e.g., Catharanthus roseus) vs. low-TIA species under controlled light conditions (e.g., red/blue light modulation of GATA transcription factors) .
- Use CRISPR-Cas9 to knockout this compound synthase in model plants and assess downstream secologanin/strictosidine accumulation .
- Conduct docking studies with UGT94F2/UGT86C4 to map substrate specificity toward this compound derivatives .
Q. How can metabolic bottlenecks in this compound production be identified and overcome in synthetic pathways?
- Apply 13C metabolic flux analysis to pinpoint rate-limiting steps (e.g., precursor supply from the MEP pathway).
- Engineer chimeric enzymes (e.g., fusion proteins linking IO and ADH) to reduce intermediate diffusion .
- Use dynamic regulation (e.g., quorum sensing-based promoters) to decouple growth phase from production phase .
Q. Methodological Considerations
Q. What criteria should guide the selection of enzyme variants for this compound pathway optimization?
Prioritize variants with:
- ≥85% sequence identity to native enzymes (e.g., CrolO) to ensure functional fidelity.
- Thermostability (assayed via differential scanning fluorimetry) for industrial scalability.
- Compatibility with host redox cofactors (e.g., NADPH/NAD+ ratios in yeast) .
Q. How can researchers ensure reproducibility in heterologous this compound production studies?
- Adhere to MIAMI guidelines for reporting metabolic engineering experiments.
- Deposit engineered strains in public repositories (e.g., Addgene).
- Include negative controls (e.g., empty vector strains) and technical triplicates in assays .
Q. Data Analysis & Interpretation
Q. What statistical approaches are suitable for analyzing enzyme kinetic data in this compound biosynthesis?
- Fit Michaelis-Menten curves using nonlinear regression (e.g., GraphPad Prism) to derive Km and Vmax.
- Compare catalytic efficiency (kcat/Km) across enzyme variants via ANOVA with post-hoc Tukey tests .
- Use principal component analysis (PCA) to cluster enzyme performance metrics across experimental conditions .
Q. How should conflicting NMR and LC-MS data on this compound purity be reconciled?
Propiedades
Fórmula molecular |
C10H14O4 |
---|---|
Peso molecular |
198.22 g/mol |
Nombre IUPAC |
(1R,4aS,7S,7aR)-1-hydroxy-7-methyl-1,4a,5,6,7,7a-hexahydrocyclopenta[c]pyran-4-carboxylic acid |
InChI |
InChI=1S/C10H14O4/c1-5-2-3-6-7(9(11)12)4-14-10(13)8(5)6/h4-6,8,10,13H,2-3H2,1H3,(H,11,12)/t5-,6+,8+,10+/m0/s1 |
Clave InChI |
DKGYTSKPMLWLEI-FIZOKRMRSA-N |
SMILES isomérico |
C[C@H]1CC[C@H]2[C@@H]1[C@@H](OC=C2C(=O)O)O |
SMILES canónico |
CC1CCC2C1C(OC=C2C(=O)O)O |
Origen del producto |
United States |
Retrosynthesis Analysis
AI-Powered Synthesis Planning: Our tool employs the Template_relevance Pistachio, Template_relevance Bkms_metabolic, Template_relevance Pistachio_ringbreaker, Template_relevance Reaxys, Template_relevance Reaxys_biocatalysis model, leveraging a vast database of chemical reactions to predict feasible synthetic routes.
One-Step Synthesis Focus: Specifically designed for one-step synthesis, it provides concise and direct routes for your target compounds, streamlining the synthesis process.
Accurate Predictions: Utilizing the extensive PISTACHIO, BKMS_METABOLIC, PISTACHIO_RINGBREAKER, REAXYS, REAXYS_BIOCATALYSIS database, our tool offers high-accuracy predictions, reflecting the latest in chemical research and data.
Strategy Settings
Precursor scoring | Relevance Heuristic |
---|---|
Min. plausibility | 0.01 |
Model | Template_relevance |
Template Set | Pistachio/Bkms_metabolic/Pistachio_ringbreaker/Reaxys/Reaxys_biocatalysis |
Top-N result to add to graph | 6 |
Feasible Synthetic Routes
Descargo de responsabilidad e información sobre productos de investigación in vitro
Tenga en cuenta que todos los artículos e información de productos presentados en BenchChem están destinados únicamente con fines informativos. Los productos disponibles para la compra en BenchChem están diseñados específicamente para estudios in vitro, que se realizan fuera de organismos vivos. Los estudios in vitro, derivados del término latino "in vidrio", involucran experimentos realizados en entornos de laboratorio controlados utilizando células o tejidos. Es importante tener en cuenta que estos productos no se clasifican como medicamentos y no han recibido la aprobación de la FDA para la prevención, tratamiento o cura de ninguna condición médica, dolencia o enfermedad. Debemos enfatizar que cualquier forma de introducción corporal de estos productos en humanos o animales está estrictamente prohibida por ley. Es esencial adherirse a estas pautas para garantizar el cumplimiento de los estándares legales y éticos en la investigación y experimentación.