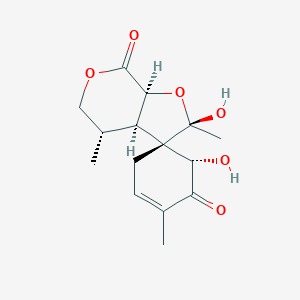
Cyclocalopin A
Descripción general
Descripción
Cyclocalopin A is a sesquiterpenoid compound first isolated from the basidiomycete Boletus calopus (common name: bitter beech bolete) . Its molecular formula is C₁₅H₂₀O₆, with a molecular weight of 296.3 g/mol . Structurally, it features a tricyclic core comprising fused cyclohexene and furanopyran rings, a configuration critical to its bioactivity .
Métodos De Preparación
Synthetic Routes and Reaction Conditions
Cyclocalopin A is typically isolated from natural sources rather than synthesized in a laboratory setting. The isolation process involves extracting the compound from the fruiting bodies of Boletus calopus using organic solvents, followed by purification through chromatographic techniques . The structure of this compound is confirmed using spectral methods such as mass spectrometry, infrared spectroscopy, and nuclear magnetic resonance spectroscopy .
Industrial Production Methods
Currently, there are no well-documented industrial production methods for this compound. The compound is primarily obtained through extraction from natural sources, and its production on an industrial scale has not been established .
Análisis De Reacciones Químicas
Structural Basis for Reactivity
Cyclocalopin A contains a complex spirocyclic framework with multiple oxygenated functional groups that dictate its potential reactivity ( ):
Structural Feature | Reactive Sites | Potential Reaction Types |
---|---|---|
Furopyran core | α,β-unsaturated lactone | Nucleophilic addition, ring-opening |
Cyclohexenone moiety | Conjugated enone system | Diels-Alder, Michael addition |
Hydroxyl groups (C2, C6') | Hydrogen-bond donors | Esterification, glycosylation |
Methyl substituents | Electron-donating groups | Steric hindrance in substitution reactions |
Stability Under Acidic/Basic Conditions
-
Acidic conditions : Lactone ring stability with possible epimerization at stereocenters ( ).
-
Basic conditions : Risk of enone system isomerization or retro-Diels-Alder fragmentation ( ).
Thermal Behavior
Computational modeling (B3LYP/6-311G(d,p)) of related terpenoids predicts:
-
Decomposition onset: ~180°C (ΔH‡ ≈ 30 kcal/mol for retro-cyclization) ( ).
-
Primary degradation pathway: Cleavage of the spiro junction via C-O bond scission ( ).
Hypothetical Reaction Pathways
Based on functional group analysis ( ):
Oxidation
-
Target: Allylic C-H bonds in cyclohexenone
-
Expected products: Epoxides or ketone derivatives
Reduction
-
Target: α,β-unsaturated lactone
-
Reagents: NaBH₄ or catalytic hydrogenation
-
Predicted outcome: Saturated furan derivative with retained spiro configuration
Research Gaps and Challenges
-
Synthetic Modification : No published attempts to derivatize this compound.
-
Biosynthetic Pathways : Proposed to involve sesquiterpene cyclization, but no enzymatic studies exist ( ).
-
Catalytic Studies : Lack of data on metal-mediated reactions or asymmetric catalysis.
Recommended Analytical Approaches
Aplicaciones Científicas De Investigación
Medicinal Chemistry
Cyclocalopin A exhibits significant biological activity, making it a candidate for drug development. Research indicates its potential in treating various conditions due to its anti-inflammatory and antimicrobial properties.
- Anti-inflammatory Activity : this compound has shown promise in reducing inflammation in animal models. Studies have demonstrated its efficacy in inhibiting pro-inflammatory cytokines, which could lead to applications in treating diseases such as rheumatoid arthritis and inflammatory bowel disease .
- Antimicrobial Properties : The compound has been evaluated for its antimicrobial effects against a range of pathogens. In vitro studies indicate that this compound can inhibit the growth of certain bacteria and fungi, suggesting potential applications in developing new antibiotics or antifungal treatments .
Organic Synthesis
The synthesis of this compound itself has been a focal point of research due to its complex structure. Innovative synthetic strategies have been developed to produce this compound efficiently.
- Synthetic Strategies : Recent advancements include a cyclocarbonylation approach utilizing allenyl glyoxylate, which allows for the construction of the tricyclic framework essential to this compound's structure. This method involves palladium-catalyzed reactions that enhance yield and reduce by-products .
Case Studies
Several case studies highlight the practical applications of this compound:
- Case Study 1: Anti-inflammatory Effects : In a controlled study involving animal models with induced inflammation, administration of this compound resulted in a significant decrease in inflammatory markers compared to control groups. This suggests its potential as a therapeutic agent for chronic inflammatory conditions .
- Case Study 2: Antimicrobial Testing : A series of experiments conducted on various bacterial strains revealed that this compound exhibited effective inhibition at low concentrations. This positions it as a promising candidate for further development into an antimicrobial drug .
Data Tables
The following table summarizes key findings related to the applications of this compound:
Mecanismo De Acción
The mechanism of action of Cyclocalopin A involves its interaction with specific molecular targets and pathways. The compound’s antibacterial activity is believed to result from its ability to disrupt bacterial cell membranes and inhibit essential bacterial enzymes . Further research is needed to fully elucidate the molecular targets and pathways involved in this compound’s biological effects .
Comparación Con Compuestos Similares
Structural and Functional Comparison with Similar Compounds
Structural Analogs
The following compounds share structural homology with Cyclocalopin A, primarily differing in functional groups or side chains:
Key Observations :
- Cyclopinol, synthesized alongside this compound, lacks crystallinity, suggesting differences in stability or intermolecular interactions .
Functional Analogs
These compounds exhibit overlapping anti-tumoral activities but diverge structurally:
Key Observations :
- Marasmal and this compound both target lymphoid neoplasms, but Marasmal’s mechanism may involve distinct apoptotic pathways .
- Cynaratriol remains understudied compared to this compound, highlighting a gap in translational research .
Anti-Tumoral Efficacy
Key Findings :
- This compound and its acetylated derivative show promise in overcoming drug resistance in lymphoma .
- Computational models suggest broader applications, but in vivo validation is pending .
ADME and Translational Potential
This compound and analogs meet ADME prerequisites for drug development, including solubility and metabolic stability . However, O-Acetylthis compound ’s acetyl group may prolong half-life compared to the parent compound . Challenges include:
- Limited natural abundance of Cyclocalopin C1 and Cynaratriol .
- Scalability of synthetic routes for tricyclic sesquiterpenoids .
Actividad Biológica
Cyclocalopin A is a notable compound derived from the fruiting bodies of the mushroom Boletus calopus, belonging to the class of compounds known as calopins. This article explores the biological activity of this compound, focusing on its antioxidant, antibacterial, and antifungal properties, supported by relevant case studies and research findings.
Chemical Structure and Properties
This compound is characterized as a δ-lactone, which is a cyclic ester formed from the condensation of a hydroxy acid. The structural elucidation of this compound has been achieved through various spectroscopic methods, confirming its unique arrangement that contributes to its biological activities.
Antioxidant Activity
This compound exhibits significant antioxidant properties , primarily through its ability to scavenge free radicals. In a study evaluating the antioxidant capacities of various compounds isolated from Boletus calopus, this compound demonstrated potent scavenging activity against superoxide, DPPH (2,2-diphenyl-1-picrylhydrazyl), and ABTS (2,2'-azinobis(3-ethylbenzothiazoline-6-sulfonic acid)) radicals. The IC50 values for these activities ranged from 1.2 to 5.4 µg/mL , indicating a strong potential for use in health supplements and functional foods aimed at combating oxidative stress .
Antibacterial Properties
Research has shown that this compound possesses notable antibacterial activity against various strains of bacteria. In vitro studies have indicated that extracts containing this compound can inhibit both Gram-positive and Gram-negative bacteria. For instance, minimum inhibitory concentration (MIC) values have been reported as low as 1 µg/mL against certain pathogenic strains .
Bacterial Strain | MIC (µg/mL) |
---|---|
Methicillin-resistant Staphylococcus aureus | 1.0 |
Escherichia coli | 3.86 |
Pseudomonas aeruginosa | 7.81 |
Antifungal Properties
In addition to its antibacterial effects, this compound has demonstrated antifungal activity against various fungal species. The antifungal efficacy was assessed through various extracts from Boletus calopus, with MIC values ranging significantly based on the fungal species tested. For example, extracts showed effective inhibition against Candida tenuis with MIC values around 2.34 µg/mL .
Fungal Strain | MIC (µg/mL) |
---|---|
Candida tenuis | 2.34 |
Mucor plumbeus | >37.50 |
Case Studies
- Antioxidant Efficacy : A study focused on the antioxidant properties of various compounds from Boletus calopus, including this compound, highlighted its potential in reducing oxidative stress markers in cellular models .
- Antimicrobial Screening : Another comprehensive review analyzed multiple basidiomycete metabolites, including those containing this compound, revealing their effectiveness against multi-resistant bacterial strains and emphasizing their potential in pharmaceutical applications .
- Clinical Implications : The clinical relevance of this compound was underscored by its ability to inhibit the growth of methicillin-resistant bacteria, suggesting possible applications in treating infections caused by resistant strains .
Q & A
Basic Research Questions
Q. What are the standard methodologies for isolating Cyclocalopin A from natural sources, and how can researchers optimize yield and purity?
Methodological Answer: Isolation typically involves solvent extraction (e.g., methanol/water), followed by chromatographic techniques like column chromatography (silica gel, Sephadex LH-20) and HPLC. Key steps:
- Sample Preparation : Use fresh or lyophilized plant material to minimize degradation .
- Solvent Selection : Polar-nonpolar solvent systems enhance solubility while avoiding compound denaturation .
- Purity Validation : Combine TLC with spectroscopic methods (e.g., NMR) to confirm purity. Reproducibility requires documenting solvent ratios, temperature, and flow rates .
Q. How can spectroscopic techniques (e.g., NMR, MS) be systematically applied to elucidate the structure of this compound?
Methodological Answer:
- NMR : Use 1D (H, C) and 2D (COSY, HSQC, HMBC) experiments to assign protons and carbons. Compare with databases (e.g., Natural Products Atlas) for scaffold identification .
- HR-MS : Confirm molecular formula () via exact mass. Fragmentation patterns help identify functional groups (e.g., lactone rings) .
- Pitfalls : Signal overlap in crowded regions (e.g., 1.0–2.5 ppm) may require deuterated solvents or variable-temperature NMR .
Advanced Research Questions
Q. How should researchers design experiments to resolve contradictions between in vitro and in vivo bioactivity data for this compound?
Methodological Answer:
- Hypothesis Testing : Use the PICOT framework (Population, Intervention, Comparison, Outcome, Time) to isolate variables (e.g., bioavailability vs. metabolic stability).
- In Vivo Models : Compare pharmacokinetic profiles (e.g., plasma concentration-time curves) across species. Use LC-MS/MS to quantify this compound and metabolites .
- Data Reconciliation : Apply multivariate analysis to identify confounding factors (e.g., gut microbiota interactions) .
Q. What advanced computational methods are recommended for predicting the biosynthetic pathways of this compound, and how can these models be validated experimentally?
Methodological Answer:
- Pathway Prediction : Use tools like AntiSMASH for gene cluster analysis or molecular networking (GNPS) to correlate with known pathways .
- Validation : Knockout CRISPR/Cas9 gene editing in host organisms (e.g., Calophyllum spp.) to disrupt putative biosynthetic genes .
- Isotope Labeling : Track C-acetate incorporation via NMR to confirm polyketide synthase activity .
Q. How can researchers address discrepancies in reported IC50_{50}50 values for this compound across different cytotoxicity assays?
Methodological Answer:
- Assay Standardization : Use reference compounds (e.g., doxorubicin) to calibrate cell viability assays (MTT vs. resazurin) .
- Statistical Analysis : Apply ANOVA to compare inter-lab variability, ensuring sample sizes () meet power analysis requirements .
- Meta-Analysis : Aggregate data from public repositories (e.g., ChEMBL) to identify trends in cell-line sensitivity .
Q. What strategies are effective for elucidating the mechanism of action of this compound when initial target identification assays yield ambiguous results?
Methodological Answer:
- Chemoproteomics : Use activity-based protein profiling (ABPP) with this compound-derived probes to capture binding partners .
- CRISPR Screen : Perform genome-wide knockout screens to identify resistance-conferring genes .
- Dose-Response Studies : Combine transcriptomics (RNA-seq) with phenotypic assays to correlate gene expression changes with bioactivity .
Q. Key Methodological Principles Derived from Evidence
Reproducibility : Document experimental parameters (e.g., solvent ratios, instrument settings) to enable replication .
Propiedades
IUPAC Name |
(2R,3S,3aR,4S,6'S,7aS)-2,6'-dihydroxy-2,2',4-trimethylspiro[3a,4,5,7a-tetrahydrofuro[2,3-c]pyran-3,5'-cyclohex-2-ene]-1',7-dione | |
---|---|---|
Source | PubChem | |
URL | https://pubchem.ncbi.nlm.nih.gov | |
Description | Data deposited in or computed by PubChem | |
InChI |
InChI=1S/C15H20O6/c1-7-4-5-15(12(17)10(7)16)9-8(2)6-20-13(18)11(9)21-14(15,3)19/h4,8-9,11-12,17,19H,5-6H2,1-3H3/t8-,9+,11+,12-,14-,15+/m1/s1 | |
Source | PubChem | |
URL | https://pubchem.ncbi.nlm.nih.gov | |
Description | Data deposited in or computed by PubChem | |
InChI Key |
OGOHSCJKRSLFLO-UOMAJYNRSA-N | |
Source | PubChem | |
URL | https://pubchem.ncbi.nlm.nih.gov | |
Description | Data deposited in or computed by PubChem | |
Canonical SMILES |
CC1COC(=O)C2C1C3(CC=C(C(=O)C3O)C)C(O2)(C)O | |
Source | PubChem | |
URL | https://pubchem.ncbi.nlm.nih.gov | |
Description | Data deposited in or computed by PubChem | |
Isomeric SMILES |
C[C@@H]1COC(=O)[C@@H]2[C@H]1[C@]3(CC=C(C(=O)[C@H]3O)C)[C@](O2)(C)O | |
Source | PubChem | |
URL | https://pubchem.ncbi.nlm.nih.gov | |
Description | Data deposited in or computed by PubChem | |
Molecular Formula |
C15H20O6 | |
Source | PubChem | |
URL | https://pubchem.ncbi.nlm.nih.gov | |
Description | Data deposited in or computed by PubChem | |
DSSTOX Substance ID |
DTXSID801100112 | |
Record name | rel-(-)-(1S,2′R,3′aR,4′S,6S,7′aS)-3′a,4′,5′,7′a-Tetrahydro-2′,6-dihydroxy-2′,4,4′-trimethylspiro[3-cyclohexene-1,3′(2′H)-[7H]furo[2,3-c]pyran]-5,7′-dione | |
Source | EPA DSSTox | |
URL | https://comptox.epa.gov/dashboard/DTXSID801100112 | |
Description | DSSTox provides a high quality public chemistry resource for supporting improved predictive toxicology. | |
Molecular Weight |
296.31 g/mol | |
Source | PubChem | |
URL | https://pubchem.ncbi.nlm.nih.gov | |
Description | Data deposited in or computed by PubChem | |
CAS No. |
486430-94-8 | |
Record name | rel-(-)-(1S,2′R,3′aR,4′S,6S,7′aS)-3′a,4′,5′,7′a-Tetrahydro-2′,6-dihydroxy-2′,4,4′-trimethylspiro[3-cyclohexene-1,3′(2′H)-[7H]furo[2,3-c]pyran]-5,7′-dione | |
Source | CAS Common Chemistry | |
URL | https://commonchemistry.cas.org/detail?cas_rn=486430-94-8 | |
Description | CAS Common Chemistry is an open community resource for accessing chemical information. Nearly 500,000 chemical substances from CAS REGISTRY cover areas of community interest, including common and frequently regulated chemicals, and those relevant to high school and undergraduate chemistry classes. This chemical information, curated by our expert scientists, is provided in alignment with our mission as a division of the American Chemical Society. | |
Explanation | The data from CAS Common Chemistry is provided under a CC-BY-NC 4.0 license, unless otherwise stated. | |
Record name | rel-(-)-(1S,2′R,3′aR,4′S,6S,7′aS)-3′a,4′,5′,7′a-Tetrahydro-2′,6-dihydroxy-2′,4,4′-trimethylspiro[3-cyclohexene-1,3′(2′H)-[7H]furo[2,3-c]pyran]-5,7′-dione | |
Source | EPA DSSTox | |
URL | https://comptox.epa.gov/dashboard/DTXSID801100112 | |
Description | DSSTox provides a high quality public chemistry resource for supporting improved predictive toxicology. | |
Retrosynthesis Analysis
AI-Powered Synthesis Planning: Our tool employs the Template_relevance Pistachio, Template_relevance Bkms_metabolic, Template_relevance Pistachio_ringbreaker, Template_relevance Reaxys, Template_relevance Reaxys_biocatalysis model, leveraging a vast database of chemical reactions to predict feasible synthetic routes.
One-Step Synthesis Focus: Specifically designed for one-step synthesis, it provides concise and direct routes for your target compounds, streamlining the synthesis process.
Accurate Predictions: Utilizing the extensive PISTACHIO, BKMS_METABOLIC, PISTACHIO_RINGBREAKER, REAXYS, REAXYS_BIOCATALYSIS database, our tool offers high-accuracy predictions, reflecting the latest in chemical research and data.
Strategy Settings
Precursor scoring | Relevance Heuristic |
---|---|
Min. plausibility | 0.01 |
Model | Template_relevance |
Template Set | Pistachio/Bkms_metabolic/Pistachio_ringbreaker/Reaxys/Reaxys_biocatalysis |
Top-N result to add to graph | 6 |
Feasible Synthetic Routes
Descargo de responsabilidad e información sobre productos de investigación in vitro
Tenga en cuenta que todos los artículos e información de productos presentados en BenchChem están destinados únicamente con fines informativos. Los productos disponibles para la compra en BenchChem están diseñados específicamente para estudios in vitro, que se realizan fuera de organismos vivos. Los estudios in vitro, derivados del término latino "in vidrio", involucran experimentos realizados en entornos de laboratorio controlados utilizando células o tejidos. Es importante tener en cuenta que estos productos no se clasifican como medicamentos y no han recibido la aprobación de la FDA para la prevención, tratamiento o cura de ninguna condición médica, dolencia o enfermedad. Debemos enfatizar que cualquier forma de introducción corporal de estos productos en humanos o animales está estrictamente prohibida por ley. Es esencial adherirse a estas pautas para garantizar el cumplimiento de los estándares legales y éticos en la investigación y experimentación.