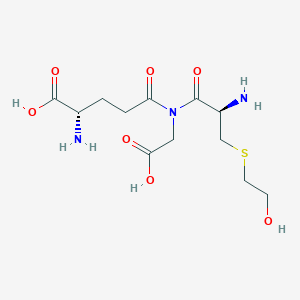
Glycine, N-(N-L-gamma-glutamyl-S-(2-hydroxyethyl)-L-cysteinyl)-
- Haga clic en CONSULTA RÁPIDA para recibir una cotización de nuestro equipo de expertos.
- Con productos de calidad a un precio COMPETITIVO, puede centrarse más en su investigación.
Descripción general
Descripción
Glycine, N-(N-L-gamma-glutamyl-S-(2-hydroxyethyl)-L-cysteinyl)-: is a complex organic compound that plays a significant role in various biochemical processes. This compound is a derivative of glycine, an amino acid that is fundamental to protein synthesis and various metabolic pathways. The structure of this compound includes glycine linked to a modified glutamyl-cysteinyl moiety, which introduces additional functional groups that can participate in diverse chemical reactions.
Métodos De Preparación
Synthetic Routes and Reaction Conditions
The synthesis of Glycine, N-(N-L-gamma-glutamyl-S-(2-hydroxyethyl)-L-cysteinyl)- typically involves multi-step organic synthesis techniques. One common method includes:
Starting Materials: Glycine, L-glutamic acid, L-cysteine, and 2-hydroxyethylamine.
Step 1: Protection of functional groups in L-glutamic acid and L-cysteine to prevent unwanted side reactions.
Step 2: Formation of the gamma-glutamyl-cysteine intermediate through peptide bond formation using coupling reagents like EDC (1-ethyl-3-(3-dimethylaminopropyl)carbodiimide) and HOBt (1-hydroxybenzotriazole).
Step 3: Introduction of the 2-hydroxyethyl group via nucleophilic substitution or addition reactions.
Step 4: Deprotection of functional groups to yield the final compound.
Industrial Production Methods
Industrial production of this compound may involve similar steps but on a larger scale, utilizing automated peptide synthesizers and optimized reaction conditions to ensure high yield and purity. Techniques such as high-performance liquid chromatography (HPLC) are employed for purification.
Análisis De Reacciones Químicas
Types of Reactions
Oxidation: The compound can undergo oxidation reactions, particularly at the cysteinyl thiol group, forming disulfides or sulfoxides.
Reduction: Reduction reactions can target any oxidized sulfur groups, reverting them to thiols.
Substitution: The hydroxyethyl group can participate in substitution reactions, where the hydroxyl group is replaced by other functional groups.
Common Reagents and Conditions
Oxidizing Agents: Hydrogen peroxide (H₂O₂), iodine (I₂).
Reducing Agents: Dithiothreitol (DTT), tris(2-carboxyethyl)phosphine (TCEP).
Substitution Reagents: Alkyl halides, acyl chlorides.
Major Products
Disulfides: Formed from oxidation of thiol groups.
Sulfoxides: Resulting from partial oxidation.
Substituted Derivatives: Depending on the substituent introduced during substitution reactions.
Aplicaciones Científicas De Investigación
Chemistry
In chemistry, this compound is used as a building block for synthesizing more complex molecules. Its unique structure allows for the exploration of new reaction pathways and the development of novel materials.
Biology
Biologically, Glycine, N-(N-L-gamma-glutamyl-S-(2-hydroxyethyl)-L-cysteinyl)- is studied for its role in cellular processes, particularly in redox reactions and as a precursor to glutathione, a critical antioxidant.
Medicine
In medicine, this compound is investigated for its potential therapeutic effects, including its role in detoxification processes and its antioxidant properties, which may help in treating oxidative stress-related conditions.
Industry
Industrially, it is used in the formulation of pharmaceuticals and nutraceuticals, leveraging its biochemical properties to enhance product efficacy.
Mecanismo De Acción
The compound exerts its effects primarily through its involvement in redox reactions. The cysteinyl thiol group can donate electrons, neutralizing reactive oxygen species (ROS) and protecting cells from oxidative damage. This activity is crucial in maintaining cellular homeostasis and preventing oxidative stress-related diseases.
Comparación Con Compuestos Similares
Similar Compounds
Glutathione: A tripeptide composed of glutamine, cysteine, and glycine, known for its antioxidant properties.
N-Acetylcysteine: A derivative of cysteine used as a mucolytic agent and antioxidant.
Uniqueness
Glycine, N-(N-L-gamma-glutamyl-S-(2-hydroxyethyl)-L-cysteinyl)- is unique due to the presence of the 2-hydroxyethyl group, which imparts additional reactivity and potential for forming diverse chemical derivatives. This structural feature distinguishes it from other similar compounds and expands its utility in various scientific and industrial applications.
Propiedades
Número CAS |
28747-20-8 |
---|---|
Fórmula molecular |
C12H21N3O7S |
Peso molecular |
351.38 g/mol |
Nombre IUPAC |
(2S)-2-amino-5-[[(2R)-1-(carboxymethylamino)-3-(2-hydroxyethylsulfanyl)-1-oxopropan-2-yl]amino]-5-oxopentanoic acid |
InChI |
InChI=1S/C12H21N3O7S/c13-7(12(21)22)1-2-9(17)15-8(6-23-4-3-16)11(20)14-5-10(18)19/h7-8,16H,1-6,13H2,(H,14,20)(H,15,17)(H,18,19)(H,21,22)/t7-,8-/m0/s1 |
Clave InChI |
UUZCUSQQEJSIHR-YUMQZZPRSA-N |
SMILES |
C(CC(=O)N(CC(=O)O)C(=O)C(CSCCO)N)C(C(=O)O)N |
SMILES isomérico |
C(CC(=O)N[C@@H](CSCCO)C(=O)NCC(=O)O)[C@@H](C(=O)O)N |
SMILES canónico |
C(CC(=O)NC(CSCCO)C(=O)NCC(=O)O)C(C(=O)O)N |
Secuencia |
XXG |
Sinónimos |
L-γ-Glutamyl-S-(2-hydroxyethyl)-L-cysteinylglycine; L-N-[1-[(Carboxymethyl)carbamoyl]-2-[(2-hydroxyethyl)thio]ethyl]glutamine; N-[N-L-γ-Glutamyl-S-(2-hydroxyethyl)-L-cysteinyl]glycine; S-(β-Hydroxyethyl)glutathione; |
Origen del producto |
United States |
Retrosynthesis Analysis
AI-Powered Synthesis Planning: Our tool employs the Template_relevance Pistachio, Template_relevance Bkms_metabolic, Template_relevance Pistachio_ringbreaker, Template_relevance Reaxys, Template_relevance Reaxys_biocatalysis model, leveraging a vast database of chemical reactions to predict feasible synthetic routes.
One-Step Synthesis Focus: Specifically designed for one-step synthesis, it provides concise and direct routes for your target compounds, streamlining the synthesis process.
Accurate Predictions: Utilizing the extensive PISTACHIO, BKMS_METABOLIC, PISTACHIO_RINGBREAKER, REAXYS, REAXYS_BIOCATALYSIS database, our tool offers high-accuracy predictions, reflecting the latest in chemical research and data.
Strategy Settings
Precursor scoring | Relevance Heuristic |
---|---|
Min. plausibility | 0.01 |
Model | Template_relevance |
Template Set | Pistachio/Bkms_metabolic/Pistachio_ringbreaker/Reaxys/Reaxys_biocatalysis |
Top-N result to add to graph | 6 |
Feasible Synthetic Routes
Descargo de responsabilidad e información sobre productos de investigación in vitro
Tenga en cuenta que todos los artículos e información de productos presentados en BenchChem están destinados únicamente con fines informativos. Los productos disponibles para la compra en BenchChem están diseñados específicamente para estudios in vitro, que se realizan fuera de organismos vivos. Los estudios in vitro, derivados del término latino "in vidrio", involucran experimentos realizados en entornos de laboratorio controlados utilizando células o tejidos. Es importante tener en cuenta que estos productos no se clasifican como medicamentos y no han recibido la aprobación de la FDA para la prevención, tratamiento o cura de ninguna condición médica, dolencia o enfermedad. Debemos enfatizar que cualquier forma de introducción corporal de estos productos en humanos o animales está estrictamente prohibida por ley. Es esencial adherirse a estas pautas para garantizar el cumplimiento de los estándares legales y éticos en la investigación y experimentación.