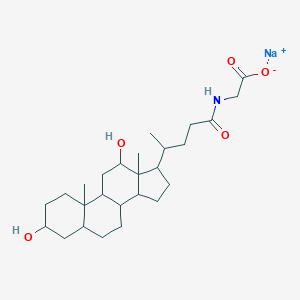
Sodium glycodeoxycholate
Descripción general
Descripción
Sodium glycodeoxycholate is a bile salt that can be used to form biomimicking environments . It is the sodium salt of glycodeoxycholic acid and has a role as a human metabolite . It has been used in a study to develop more similar mimetic intestinal fluids to physiological fluids than those developed by mixed bile salt systems (MBS) .
Synthesis Analysis
Sodium glycodeoxycholate is synthesized from cholesterol in the liver . The synthesis of bile salts is the major route for elimination of cholesterol from the body .Molecular Structure Analysis
The structure of Sodium glycodeoxycholate consists of four rings, three six carbon rings (A, B, and C) and one five carbon ring (D). The concave (α) side of the steroid skeleton of bile acid molecules is hydrophilic due to the presence of OH groups, while the convex (β) side with its angular methyl groups is hydrophobic .Chemical Reactions Analysis
Sodium glycodeoxycholate acts as a detergent to solubilize fats for absorption and is itself absorbed . It can induce apoptosis in hepatocytes and may induce DNA cleavage .Physical And Chemical Properties Analysis
Sodium glycodeoxycholate is an anionic compound with a molecular weight of 471.61 g/mol . It has a solubility of 0.1 M in water, forming a clear to slightly hazy, colorless to almost colorless solution .Aplicaciones Científicas De Investigación
Enhancing Buccal Delivery of Insulin
Sodium Glycodeoxycholate has been used in the development of elastic liposomes to enhance the buccal delivery of insulin . The Sodium Glycodeoxycholate-incorporated elastic liposomes (SGDC-EL) were found to significantly enhance the permeation of insulin across porcine buccal tissues . This suggests that Sodium Glycodeoxycholate could be used to improve the effectiveness of buccal drug delivery systems .
Forming Biomimicking Environments
Sodium Glycodeoxycholate is a bile salt that can be used to form biomimicking environments . This could have potential applications in the development of more physiologically relevant in vitro models for drug testing and other biomedical research .
Development of Mimetic Intestinal Fluids
Sodium Glycodeoxycholate has been used in a study to develop more similar mimetic intestinal fluids to physiological fluids than those developed by mixed bile salt systems (MBS) . This could be useful in the development of more accurate in vitro models for studying intestinal absorption and metabolism .
Investigation of Ca2+ Buffering Capability of Bile Salt Micelles
Sodium Glycodeoxycholate has also been used in a study to investigate the Ca2+ buffering capability of bile salt micelles . This could have implications for understanding the role of bile salts in calcium homeostasis .
Interaction with Dietary Fibres
Dietary fibres are reported to interact with bile acids, preventing their reabsorption and promoting their excretion into the colon . Sodium Glycodeoxycholate, being a bile salt, could potentially be used to study these interactions and their implications for health .
Effects on Cell Viability
Sodium Glycodeoxycholate has been found to have dual effects on cell viability, increasing it at lower concentrations and decreasing it at higher ones . This could have potential applications in the development of therapeutic strategies for diseases where cell viability is a key factor .
Mecanismo De Acción
Target of Action
Sodium glycodeoxycholate primarily targets hepatocytes . Hepatocytes are the main cell type in the liver and play a crucial role in protein synthesis, metabolism, and detoxification .
Mode of Action
Sodium glycodeoxycholate interacts with hepatocytes and induces apoptosis , a process of programmed cell death . This process is associated with DNA cleavage by endonucleases . Endonucleases are enzymes that cleave the phosphodiester bond within a polynucleotide chain, facilitating the breakdown of DNA.
Biochemical Pathways
Sodium glycodeoxycholate is a secondary bile acid produced by the action of enzymes existing in the microbial flora of the colonic environment . Bile acids are crucial for the digestion and absorption of dietary fats in the small intestine. They form micelles, which are tiny clusters of bile salts that transport lipids across the cell membrane .
Pharmacokinetics
The pharmacokinetics of sodium glycodeoxycholate involve its absorption and distribution in the body. It has been studied as an absorption enhancer to increase drug transport across various biological barriers such as the blood-brain barrier, skin, mucosa, cornea, buccal, nasal, pulmonary, and intestinal membranes . It enhances absorption by increasing the solubility of hydrophobic drugs or by increasing the fluidity of the apical and basolateral membranes .
Result of Action
The primary result of sodium glycodeoxycholate’s action is the induction of apoptosis in hepatocytes . This can lead to the breakdown of DNA, contributing to the programmed cell death process .
Action Environment
The action of sodium glycodeoxycholate can be influenced by various environmental factors. For instance, its efficacy as an absorption enhancer can be affected by the physiological conditions of the biological barriers it interacts with . Furthermore, its stability and efficacy can be influenced by factors such as pH and the presence of other compounds .
Direcciones Futuras
Sodium glycodeoxycholate has been used in studies to develop more similar mimetic intestinal fluids to physiological fluids than those developed by mixed bile salt systems (MBS) . It has also been used in a study to investigate Ca 2+ buffering capability of bile salt micelles . Future research could focus on combining stem cells and biomaterials for clinical treatment in stomatology .
Propiedades
IUPAC Name |
sodium;2-[[(4R)-4-[(3R,5R,8R,9S,10S,12S,13R,14S,17R)-3,12-dihydroxy-10,13-dimethyl-2,3,4,5,6,7,8,9,11,12,14,15,16,17-tetradecahydro-1H-cyclopenta[a]phenanthren-17-yl]pentanoyl]amino]acetate | |
---|---|---|
Source | PubChem | |
URL | https://pubchem.ncbi.nlm.nih.gov | |
Description | Data deposited in or computed by PubChem | |
InChI |
InChI=1S/C26H43NO5.Na/c1-15(4-9-23(30)27-14-24(31)32)19-7-8-20-18-6-5-16-12-17(28)10-11-25(16,2)21(18)13-22(29)26(19,20)3;/h15-22,28-29H,4-14H2,1-3H3,(H,27,30)(H,31,32);/q;+1/p-1/t15-,16-,17-,18+,19-,20+,21+,22+,25+,26-;/m1./s1 | |
Source | PubChem | |
URL | https://pubchem.ncbi.nlm.nih.gov | |
Description | Data deposited in or computed by PubChem | |
InChI Key |
VMSNAUAEKXEYGP-YEUHZSMFSA-M | |
Source | PubChem | |
URL | https://pubchem.ncbi.nlm.nih.gov | |
Description | Data deposited in or computed by PubChem | |
Canonical SMILES |
CC(CCC(=O)NCC(=O)[O-])C1CCC2C1(C(CC3C2CCC4C3(CCC(C4)O)C)O)C.[Na+] | |
Source | PubChem | |
URL | https://pubchem.ncbi.nlm.nih.gov | |
Description | Data deposited in or computed by PubChem | |
Isomeric SMILES |
C[C@H](CCC(=O)NCC(=O)[O-])[C@H]1CC[C@@H]2[C@@]1([C@H](C[C@H]3[C@H]2CC[C@H]4[C@@]3(CC[C@H](C4)O)C)O)C.[Na+] | |
Source | PubChem | |
URL | https://pubchem.ncbi.nlm.nih.gov | |
Description | Data deposited in or computed by PubChem | |
Molecular Formula |
C26H42NNaO5 | |
Source | PubChem | |
URL | https://pubchem.ncbi.nlm.nih.gov | |
Description | Data deposited in or computed by PubChem | |
Molecular Weight |
471.6 g/mol | |
Source | PubChem | |
URL | https://pubchem.ncbi.nlm.nih.gov | |
Description | Data deposited in or computed by PubChem | |
Product Name |
Sodium glycodeoxycholate | |
CAS RN |
16409-34-0 | |
Record name | Sodium glycodeoxycholate | |
Source | ChemIDplus | |
URL | https://pubchem.ncbi.nlm.nih.gov/substance/?source=chemidplus&sourceid=0016409340 | |
Description | ChemIDplus is a free, web search system that provides access to the structure and nomenclature authority files used for the identification of chemical substances cited in National Library of Medicine (NLM) databases, including the TOXNET system. | |
Record name | Sodium N-[(3α,5β,12α)-3,12-dihydroxy-24-oxocholan-24-yl]aminoacetate | |
Source | European Chemicals Agency (ECHA) | |
URL | https://echa.europa.eu/substance-information/-/substanceinfo/100.036.762 | |
Description | The European Chemicals Agency (ECHA) is an agency of the European Union which is the driving force among regulatory authorities in implementing the EU's groundbreaking chemicals legislation for the benefit of human health and the environment as well as for innovation and competitiveness. | |
Explanation | Use of the information, documents and data from the ECHA website is subject to the terms and conditions of this Legal Notice, and subject to other binding limitations provided for under applicable law, the information, documents and data made available on the ECHA website may be reproduced, distributed and/or used, totally or in part, for non-commercial purposes provided that ECHA is acknowledged as the source: "Source: European Chemicals Agency, http://echa.europa.eu/". Such acknowledgement must be included in each copy of the material. ECHA permits and encourages organisations and individuals to create links to the ECHA website under the following cumulative conditions: Links can only be made to webpages that provide a link to the Legal Notice page. | |
Record name | SODIUM GLYCODEOXYCHOLATE | |
Source | FDA Global Substance Registration System (GSRS) | |
URL | https://gsrs.ncats.nih.gov/ginas/app/beta/substances/6W76V75MT1 | |
Description | The FDA Global Substance Registration System (GSRS) enables the efficient and accurate exchange of information on what substances are in regulated products. Instead of relying on names, which vary across regulatory domains, countries, and regions, the GSRS knowledge base makes it possible for substances to be defined by standardized, scientific descriptions. | |
Explanation | Unless otherwise noted, the contents of the FDA website (www.fda.gov), both text and graphics, are not copyrighted. They are in the public domain and may be republished, reprinted and otherwise used freely by anyone without the need to obtain permission from FDA. Credit to the U.S. Food and Drug Administration as the source is appreciated but not required. | |
Retrosynthesis Analysis
AI-Powered Synthesis Planning: Our tool employs the Template_relevance Pistachio, Template_relevance Bkms_metabolic, Template_relevance Pistachio_ringbreaker, Template_relevance Reaxys, Template_relevance Reaxys_biocatalysis model, leveraging a vast database of chemical reactions to predict feasible synthetic routes.
One-Step Synthesis Focus: Specifically designed for one-step synthesis, it provides concise and direct routes for your target compounds, streamlining the synthesis process.
Accurate Predictions: Utilizing the extensive PISTACHIO, BKMS_METABOLIC, PISTACHIO_RINGBREAKER, REAXYS, REAXYS_BIOCATALYSIS database, our tool offers high-accuracy predictions, reflecting the latest in chemical research and data.
Strategy Settings
Precursor scoring | Relevance Heuristic |
---|---|
Min. plausibility | 0.01 |
Model | Template_relevance |
Template Set | Pistachio/Bkms_metabolic/Pistachio_ringbreaker/Reaxys/Reaxys_biocatalysis |
Top-N result to add to graph | 6 |
Feasible Synthetic Routes
Descargo de responsabilidad e información sobre productos de investigación in vitro
Tenga en cuenta que todos los artículos e información de productos presentados en BenchChem están destinados únicamente con fines informativos. Los productos disponibles para la compra en BenchChem están diseñados específicamente para estudios in vitro, que se realizan fuera de organismos vivos. Los estudios in vitro, derivados del término latino "in vidrio", involucran experimentos realizados en entornos de laboratorio controlados utilizando células o tejidos. Es importante tener en cuenta que estos productos no se clasifican como medicamentos y no han recibido la aprobación de la FDA para la prevención, tratamiento o cura de ninguna condición médica, dolencia o enfermedad. Debemos enfatizar que cualquier forma de introducción corporal de estos productos en humanos o animales está estrictamente prohibida por ley. Es esencial adherirse a estas pautas para garantizar el cumplimiento de los estándares legales y éticos en la investigación y experimentación.