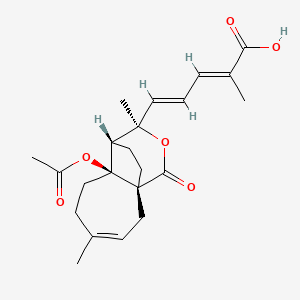
Pseudolaric acid A
Descripción general
Descripción
Mecanismo De Acción
Target of Action
Pseudolaric Acid A (PAA) is a bioactive ingredient in traditional medicine Pseudolarix cortex . The primary target of PAA is Hsp90 , a heat shock protein . Hsp90 is a molecular chaperone involved in the stability and function of other proteins. PAA directly binds to Hsp90, inhibiting its function .
Mode of Action
PAA interacts with Hsp90, leading to the disruption of its function . This interaction induces cell cycle arrest at the G2/M phase and promotes cell death through the caspase-8/caspase-3 pathway . The binding of PAA to Hsp90 has been confirmed through multiple biophysical experiments .
Biochemical Pathways
The primary biochemical pathway affected by PAA is the cell cycle . PAA induces cell cycle arrest at the G2/M phase . This arrest is a result of the inhibition of Hsp90, which is crucial for cell cycle progression. The caspase-8/caspase-3 pathway, a part of the apoptosis mechanism, is also activated, leading to cell death .
Result of Action
The result of PAA’s action is potent antiproliferation and anticancer activities . By inducing cell cycle arrest and promoting cell death, PAA exhibits remarkable anticancer activities . The correlation between its Hsp90 inhibitory activity and anticancer activity has been revealed through structure-activity relationship studies .
Action Environment
It is known that paa is a bioactive ingredient in traditional medicine pseudolarix cortex , suggesting that it is stable and effective in the natural environment of this plant. The influence of other environmental factors on PAA’s action, efficacy, and stability is currently unclear and warrants further investigation.
Análisis Bioquímico
Biochemical Properties
Pseudolaric acid A plays a crucial role in various biochemical reactions. It interacts with several enzymes, proteins, and other biomolecules, influencing their activity and function. One notable interaction is with heat shock protein 90 (Hsp90), where this compound acts as an inhibitor. This interaction disrupts the protein’s function, leading to the inhibition of cell proliferation and induction of apoptosis in cancer cells . Additionally, this compound has been shown to interact with microtubules, destabilizing their structure and inhibiting mitotic spindle formation . These interactions highlight the compound’s potential as a therapeutic agent in cancer treatment.
Cellular Effects
This compound exerts significant effects on various cell types and cellular processes. In cancer cells, it induces cell cycle arrest at the G2/M phase and promotes apoptosis through the caspase-8/caspase-3 pathway . This compound also affects cell signaling pathways, such as the nuclear factor kappa-B (NF-κB) pathway, leading to reduced expression of inflammatory cytokines . Furthermore, this compound influences gene expression and cellular metabolism, contributing to its antiproliferative and anticancer activities.
Molecular Mechanism
The molecular mechanism of this compound involves several key interactions at the molecular level. As an Hsp90 inhibitor, this compound binds to the N-terminal domain of Hsp90, disrupting its chaperone function and leading to the degradation of client proteins . This inhibition results in the suppression of cell growth and induction of apoptosis. Additionally, this compound targets microtubules, destabilizing their structure and preventing proper mitotic spindle formation . These molecular interactions underscore the compound’s potential as a therapeutic agent in cancer treatment.
Temporal Effects in Laboratory Settings
In laboratory settings, the effects of this compound have been observed to change over time. The compound exhibits stability under various conditions, maintaining its bioactivity over extended periods. Degradation can occur under certain conditions, leading to a reduction in its efficacy . Long-term studies have shown that this compound can induce sustained changes in cellular function, including prolonged cell cycle arrest and apoptosis . These findings highlight the importance of understanding the temporal dynamics of this compound’s effects in laboratory settings.
Dosage Effects in Animal Models
The effects of this compound vary with different dosages in animal models. At lower doses, the compound exhibits potent anticancer activity with minimal toxicity . At higher doses, this compound can induce toxic effects, including hepatotoxicity and nephrotoxicity . These findings underscore the importance of determining the optimal dosage for therapeutic applications to maximize efficacy while minimizing adverse effects.
Metabolic Pathways
This compound is involved in several metabolic pathways, interacting with various enzymes and cofactors. The compound is metabolized primarily in the liver, where it undergoes biotransformation by cytochrome P450 enzymes . This metabolism results in the formation of several metabolites, which can influence the compound’s bioactivity and toxicity. Additionally, this compound affects metabolic flux and metabolite levels, contributing to its overall pharmacological profile.
Transport and Distribution
Within cells and tissues, this compound is transported and distributed through various mechanisms. The compound interacts with transporters and binding proteins, facilitating its uptake and distribution . This compound can accumulate in specific tissues, such as the liver and kidneys, where it exerts its biological effects. Understanding the transport and distribution of this compound is crucial for optimizing its therapeutic potential.
Subcellular Localization
This compound exhibits specific subcellular localization, which influences its activity and function. The compound is primarily localized in the cytoplasm, where it interacts with microtubules and other cellular structures . Additionally, this compound can be directed to specific compartments or organelles through targeting signals and post-translational modifications . These localization patterns are essential for understanding the compound’s mechanism of action and optimizing its therapeutic applications.
Métodos De Preparación
Synthetic Routes and Reaction Conditions: The synthesis of pseudolaric acid A involves multiple steps, including Claisen rearrangement, iodoetherification, and ring-closing metathesis to construct its complex tricyclic core structure . The synthetic route typically starts from a known ketone and involves 17 steps to achieve the final product .
Industrial Production Methods: Industrial production of this compound is primarily based on extraction from the root bark of Pseudolarix amabilis. High-speed counter-current chromatography is often used to isolate this compound and its glucosides from the methanol extract of the root bark .
Análisis De Reacciones Químicas
Types of Reactions: Pseudolaric acid A undergoes various chemical reactions, including oxidation, reduction, and substitution reactions.
Common Reagents and Conditions:
Oxidation: Common oxidizing agents such as potassium permanganate or chromium trioxide can be used.
Reduction: Reducing agents like lithium aluminum hydride or sodium borohydride are typically employed.
Substitution: Halogenation reactions can be carried out using reagents like bromine or chlorine under controlled conditions.
Major Products: The major products formed from these reactions include various oxidized, reduced, or substituted derivatives of this compound, which can be further studied for their biological activities .
Aplicaciones Científicas De Investigación
Pseudolaric acid A has a wide range of scientific research applications:
Comparación Con Compuestos Similares
Pseudolaric acid B: Another diterpenoid from the same plant, known for its antifungal and anticancer properties.
Taxol: A well-known diterpenoid with significant anticancer activity, used in chemotherapy.
Comparison: Pseudolaric acid A is unique due to its specific inhibition of Hsp90, which is not a common target for many other diterpenoids . Unlike taxol, which stabilizes microtubules, this compound disrupts protein folding processes essential for cancer cell survival .
This compound stands out for its multifaceted biological activities and potential therapeutic applications, making it a compound of significant interest in scientific research and drug development.
Propiedades
Número CAS |
82508-32-5 |
---|---|
Fórmula molecular |
C22H28O6 |
Peso molecular |
388.5 g/mol |
Nombre IUPAC |
5-[(1R,7R,9S)-7-acetyloxy-4,9-dimethyl-11-oxo-10-oxatricyclo[6.3.2.01,7]tridec-3-en-9-yl]-2-methylpenta-2,4-dienoic acid |
InChI |
InChI=1S/C22H28O6/c1-14-7-11-21-12-9-17(22(21,13-8-14)27-16(3)23)20(4,28-19(21)26)10-5-6-15(2)18(24)25/h5-7,10,17H,8-9,11-13H2,1-4H3,(H,24,25)/t17?,20-,21+,22+/m0/s1 |
Clave InChI |
GOHMRMDXUXWCDQ-ADDXWAJHSA-N |
SMILES |
CC1=CCC23CCC(C2(CC1)OC(=O)C)C(OC3=O)(C)C=CC=C(C)C(=O)O |
SMILES isomérico |
CC1=CC[C@]23CCC([C@@]2(CC1)OC(=O)C)[C@](OC3=O)(C)C=CC=C(C)C(=O)O |
SMILES canónico |
CC1=CCC23CCC(C2(CC1)OC(=O)C)C(OC3=O)(C)C=CC=C(C)C(=O)O |
Pictogramas |
Acute Toxic; Health Hazard |
Sinónimos |
pseudolaric acid A |
Origen del producto |
United States |
Retrosynthesis Analysis
AI-Powered Synthesis Planning: Our tool employs the Template_relevance Pistachio, Template_relevance Bkms_metabolic, Template_relevance Pistachio_ringbreaker, Template_relevance Reaxys, Template_relevance Reaxys_biocatalysis model, leveraging a vast database of chemical reactions to predict feasible synthetic routes.
One-Step Synthesis Focus: Specifically designed for one-step synthesis, it provides concise and direct routes for your target compounds, streamlining the synthesis process.
Accurate Predictions: Utilizing the extensive PISTACHIO, BKMS_METABOLIC, PISTACHIO_RINGBREAKER, REAXYS, REAXYS_BIOCATALYSIS database, our tool offers high-accuracy predictions, reflecting the latest in chemical research and data.
Strategy Settings
Precursor scoring | Relevance Heuristic |
---|---|
Min. plausibility | 0.01 |
Model | Template_relevance |
Template Set | Pistachio/Bkms_metabolic/Pistachio_ringbreaker/Reaxys/Reaxys_biocatalysis |
Top-N result to add to graph | 6 |
Feasible Synthetic Routes
Q1: What is Pseudolaric Acid A (PAA) and where is it found?
A1: this compound (PAA) is a diterpenoid compound primarily isolated from the root bark of the Chinese tree Pseudolarix kaempferi Gordon (Pinaceae) [, , , , , , ].
Q2: What are the traditional medicinal uses of Pseudolarix kaempferi?
A2: Pseudolarix kaempferi has been traditionally utilized in Chinese medicine as an antifungal remedy, particularly for skin diseases caused by fungal infections [, , , ].
Q3: Does PAA possess any notable biological activities?
A3: Yes, PAA demonstrates antifungal and cytotoxic properties. It also exhibits activity against certain drug-resistant tumor cell lines [, , , , , , , , , , ].
Q4: Is PAA effective against non-albicans Candida (NAC) species?
A4: Yes, PAA exhibits antifungal activity against prevalent NAC species such as C. tropicalis, C. parapsilosis complex, and C. glabrata [].
Q5: How does PAA impact Candida albicans biofilms?
A5: PAA alone inhibits Candida albicans biofilms and shows synergistic effects when combined with fluconazole. It disrupts biofilm formation by inhibiting adhesion and the yeast-to-hypha transition [].
Q6: Does PAA demonstrate synergistic effects with other antifungals?
A6: Yes, PAA displays a synergistic antifungal effect when combined with fluconazole, particularly against fluconazole-resistant C. tropicalis [].
Q7: How does PAA affect the cell structure of C. tropicalis?
A7: PAA causes detrimental effects on the cell membranes of C. tropicalis in a dose-dependent manner [].
Q8: What intracellular changes are observed in C. tropicalis upon PAA exposure?
A8: PAA exposure leads to increased vacuole formation and the development of autophagosomes within C. tropicalis cells [].
Q9: Does PAA induce autophagy in other cell types?
A9: Yes, PAA has been shown to induce autophagy in murine fibrosarcoma L929 cells, SW579 human thyroid squamous cell carcinoma cells, and MRC5 human lung fibroblast cells [, ].
Q10: What is known about the mechanism of action of PAA?
A10: PAA's mechanism of action involves multiple pathways. It disrupts ergosterol biosynthesis in fungi [], induces cell cycle arrest and apoptosis in various cancer cell lines [, , ], and activates protein kinase C (PKC) signaling, leading to autophagy and senescence in some cell types [].
Q11: Does PAA's activity vary depending on the type of cancer cell line?
A11: Yes, PAA induces apoptosis in various cancer cell lines, but in some cell lines, like L929 murine fibrosarcoma and SW579 human thyroid squamous cell carcinoma cells, it primarily induces autophagy [, ].
Q12: Does PAA interact with the death receptor pathway to induce apoptosis?
A12: While PAB induces apoptosis through death receptor 5 (DR5) in head and neck cancer cell lines, in MCF-7 cells, PAB-induced apoptosis appears to be independent of the Fas receptor pathway [, ].
Q13: How does the structure of PAA relate to its activity?
A13: Modifying PAA's structure, such as deacetylation or esterification, can significantly alter its activity. For instance, deacetyl Pseudolaric Acid B (PLAC) and Pseudolaric Acid B-O-β-D-glucoside (PLAG) show reduced or abolished activation of PPAR isoforms compared to PAA [].
Q14: Does PAA interact with peroxisome proliferator-activated receptors (PPARs)?
A14: Yes, PAA acts as a PPAR agonist, activating PPARα, PPARγ, and PPARδ isoforms [].
Q15: What is the molecular formula and weight of PAA?
A15: The molecular formula of PAA is C22H28O6, and its molecular weight is 388.46 g/mol [, ].
Q16: What spectroscopic techniques have been employed to elucidate the structure of PAA?
A16: Various spectroscopic methods, including IR, 1H NMR, 13C NMR, and X-ray diffraction, have been used to determine the structure of PAA and its derivatives [, , , , ].
Q17: Are there established analytical methods for quantifying PAA?
A17: Yes, high-performance liquid chromatography (HPLC) methods have been developed for the quantitative analysis of PAA and related diterpenoids in plant materials and formulations [, ].
Q18: Have mass spectrometry techniques been used to study PAA?
A18: Yes, mass spectrometry, including high-resolution mass spectrometry and metastable ion analysis, has been used to study the fragmentation patterns and elucidate the structures of PAA and its derivatives [].
Q19: Have there been successful attempts to synthesize PAA?
A19: Yes, several total syntheses of PAA have been achieved, showcasing the complexity of its structure and the ingenuity of synthetic organic chemistry [, , , ].
Q20: What key synthetic strategies have been employed in the total synthesis of PAA?
A20: Key strategies in PAA synthesis include samarium diiodide-mediated intramolecular alkene-ketyl radical cyclization [], ring-closing metathesis (RCM) reactions [, ], and rhodium-mediated cycloadditions [].
Q21: What is known about the pharmacokinetics of PAA?
A21: While detailed pharmacokinetic data is limited, studies suggest that PAA undergoes metabolism, including hydrolysis and glucosylation. Pseudolaric Acid C2 appears to be the predominant metabolite detected in plasma, urine, bile, and feces [].
Descargo de responsabilidad e información sobre productos de investigación in vitro
Tenga en cuenta que todos los artículos e información de productos presentados en BenchChem están destinados únicamente con fines informativos. Los productos disponibles para la compra en BenchChem están diseñados específicamente para estudios in vitro, que se realizan fuera de organismos vivos. Los estudios in vitro, derivados del término latino "in vidrio", involucran experimentos realizados en entornos de laboratorio controlados utilizando células o tejidos. Es importante tener en cuenta que estos productos no se clasifican como medicamentos y no han recibido la aprobación de la FDA para la prevención, tratamiento o cura de ninguna condición médica, dolencia o enfermedad. Debemos enfatizar que cualquier forma de introducción corporal de estos productos en humanos o animales está estrictamente prohibida por ley. Es esencial adherirse a estas pautas para garantizar el cumplimiento de los estándares legales y éticos en la investigación y experimentación.