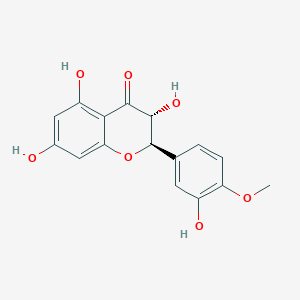
Dihidrotamarixetina
Descripción general
Descripción
Linvencorvir es un novedoso modulador de la ensambladura de la cápside (CAM) de molécula pequeña desarrollado para el tratamiento de la infección crónica por el virus de la hepatitis B (VHB). Funciona al dirigirse a la proteína central del VHB, que juega un papel crucial en el ciclo de replicación viral . El compuesto ha mostrado promesa en ensayos clínicos, particularmente en su capacidad para suprimir los niveles de ADN y ARN del VHB .
Aplicaciones Científicas De Investigación
Linvencorvir tiene una amplia gama de aplicaciones de investigación científica, que incluyen:
Química: Utilizado como un compuesto modelo para estudiar la modulación de la ensambladura de la cápside y sus efectos en la replicación viral.
Biología: Investigado por su papel en la interrupción del ciclo de vida del VHB y su potencial para lograr una cura funcional para la hepatitis B crónica.
Medicina: En ensayos clínicos para evaluar su eficacia y seguridad en el tratamiento de la infección por VHB.
Mecanismo De Acción
Linvencorvir ejerce sus efectos al dirigirse a la proteína central del VHB, un componente crítico en el proceso de replicación viral. El compuesto actúa como un modulador de la ensambladura de la cápside, interrumpiendo la formación de la cápside viral y, por lo tanto, inhibiendo la replicación del virus. Este mecanismo involucra la unión a la proteína central y la alteración de su conformación, previniendo la ensambladura adecuada de la cápside viral .
Análisis Bioquímico
Biochemical Properties
Dihydrotamarixetin plays a significant role in various biochemical reactions. It interacts with several enzymes, proteins, and other biomolecules, influencing their activity and stability. One of the primary interactions of dihydrotamarixetin is with the enzyme alcohol dehydrogenase, where it acts as an inhibitor. This interaction is crucial in modulating the enzyme’s activity, thereby affecting the metabolic pathways involving alcohols and aldehydes . Additionally, dihydrotamarixetin has been shown to interact with proteins involved in oxidative stress responses, enhancing their antioxidant capabilities and protecting cells from oxidative damage .
Cellular Effects
Dihydrotamarixetin exerts various effects on different types of cells and cellular processes. It has been observed to influence cell signaling pathways, gene expression, and cellular metabolism. In particular, dihydrotamarixetin modulates the activity of key signaling pathways such as the NF-κB pathway, which is involved in inflammatory responses . By inhibiting this pathway, dihydrotamarixetin reduces inflammation and promotes cellular homeostasis. Furthermore, dihydrotamarixetin affects gene expression by upregulating antioxidant genes and downregulating pro-inflammatory genes, thereby enhancing the cell’s ability to cope with oxidative stress .
Molecular Mechanism
The molecular mechanism of dihydrotamarixetin involves several key interactions at the molecular level. Dihydrotamarixetin binds to specific biomolecules, such as enzymes and receptors, altering their activity and function. One notable interaction is with the enzyme alcohol dehydrogenase, where dihydrotamarixetin acts as a competitive inhibitor, binding to the active site and preventing the enzyme from catalyzing its substrate . Additionally, dihydrotamarixetin has been shown to activate antioxidant response elements (AREs) in the genome, leading to the upregulation of genes involved in antioxidant defense . This activation is mediated through the binding of dihydrotamarixetin to transcription factors such as Nrf2, which then translocate to the nucleus and initiate gene transcription .
Temporal Effects in Laboratory Settings
In laboratory settings, the effects of dihydrotamarixetin have been observed to change over time. The stability of dihydrotamarixetin is relatively high, allowing it to maintain its activity over extended periods . Degradation can occur under certain conditions, such as exposure to light and high temperatures, leading to a reduction in its effectiveness . Long-term studies have shown that dihydrotamarixetin can have sustained effects on cellular function, particularly in enhancing antioxidant defenses and reducing inflammation . These effects are consistent in both in vitro and in vivo studies, highlighting the compound’s potential for long-term therapeutic applications .
Dosage Effects in Animal Models
The effects of dihydrotamarixetin vary with different dosages in animal models. At low doses, dihydrotamarixetin has been shown to exert beneficial effects, such as reducing oxidative stress and inflammation . At higher doses, toxic or adverse effects may occur, including hepatotoxicity and gastrointestinal disturbances . Threshold effects have been observed, where the beneficial effects plateau at a certain dosage, and further increases in dosage do not result in additional benefits . These findings underscore the importance of determining the optimal dosage for therapeutic applications of dihydrotamarixetin .
Metabolic Pathways
Dihydrotamarixetin is involved in several metabolic pathways, interacting with various enzymes and cofactors. One of the primary pathways is the oxidative stress response pathway, where dihydrotamarixetin enhances the activity of antioxidant enzymes such as superoxide dismutase and catalase . Additionally, dihydrotamarixetin influences the metabolism of alcohols and aldehydes by inhibiting alcohol dehydrogenase, thereby modulating the levels of these metabolites in the body . The compound also affects metabolic flux, leading to changes in the levels of key metabolites involved in energy production and cellular homeostasis .
Transport and Distribution
Within cells and tissues, dihydrotamarixetin is transported and distributed through various mechanisms. It interacts with transporters and binding proteins that facilitate its movement across cellular membranes . Once inside the cell, dihydrotamarixetin can accumulate in specific compartments, such as the cytoplasm and nucleus, where it exerts its effects . The localization and accumulation of dihydrotamarixetin are influenced by factors such as its chemical structure and the presence of specific transporters .
Subcellular Localization
The subcellular localization of dihydrotamarixetin plays a crucial role in its activity and function. Dihydrotamarixetin is primarily localized in the cytoplasm and nucleus, where it interacts with various biomolecules to exert its effects . Targeting signals and post-translational modifications may direct dihydrotamarixetin to specific compartments or organelles, enhancing its efficacy . For example, the binding of dihydrotamarixetin to transcription factors such as Nrf2 facilitates its translocation to the nucleus, where it activates antioxidant response elements and promotes gene expression .
Métodos De Preparación
Rutas sintéticas y condiciones de reacción
La síntesis de Linvencorvir involucra múltiples pasos, incluida la formación de intermedios clave y sus reacciones de acoplamiento subsecuentes. La ruta sintética exacta y las condiciones de reacción son propietarias y no se han divulgado completamente en el dominio público. Se sabe que el compuesto se sintetiza a través de una serie de reacciones orgánicas que involucran el uso de varios reactivos y catalizadores .
Métodos de producción industrial
La producción industrial de Linvencorvir probablemente involucre síntesis a gran escala utilizando condiciones de reacción optimizadas para garantizar un alto rendimiento y pureza. El proceso incluiría medidas rigurosas de control de calidad para cumplir con los estándares farmacéuticos. Los detalles específicos sobre los métodos de producción industrial no están disponibles públicamente .
Análisis De Reacciones Químicas
Tipos de reacciones
Linvencorvir experimenta varios tipos de reacciones químicas, que incluyen:
Oxidación: El compuesto se puede oxidar en condiciones específicas para formar varios derivados oxidados.
Reducción: Las reacciones de reducción se pueden usar para modificar los grupos funcionales dentro de la molécula.
Sustitución: Linvencorvir puede sufrir reacciones de sustitución donde un grupo funcional es reemplazado por otro.
Reactivos y condiciones comunes
Los reactivos comunes utilizados en las reacciones que involucran a Linvencorvir incluyen agentes oxidantes como el permanganato de potasio, agentes reductores como el borohidruro de sodio y varios nucleófilos para reacciones de sustitución. Las condiciones para estas reacciones varían según el resultado deseado, pero normalmente involucran temperaturas y niveles de pH controlados .
Productos principales formados
Los productos principales formados a partir de estas reacciones incluyen varios derivados de Linvencorvir que retienen la estructura central pero tienen grupos funcionales modificados. Estos derivados a menudo se estudian por sus posibles beneficios terapéuticos y sus propiedades farmacocinéticas mejoradas .
Comparación Con Compuestos Similares
Linvencorvir se compara con otros moduladores de la ensambladura de la cápside como vebicorvir, JNJ-6379 y JNJ-0440. Si bien estos compuestos también se dirigen a la proteína central del VHB, Linvencorvir ha mostrado propiedades únicas en ensayos clínicos, particularmente en su capacidad para suprimir los niveles de ARN del VHB . Al igual que otros CAM, tiene limitaciones para lograr una cura funcional, lo que requiere una terapia combinada con otros agentes antivirales .
Lista de compuestos similares
- Vebicorvir
- JNJ-6379
- JNJ-0440
Conclusión
Linvencorvir representa un avance prometedor en el tratamiento de la hepatitis B crónica, con su mecanismo de acción único y su potencial para interrumpir el ciclo de replicación viral. La investigación y los ensayos clínicos en curso aclararán aún más su eficacia y seguridad, allanando el camino para nuevas estrategias terapéuticas en la lucha contra la infección por VHB.
Propiedades
IUPAC Name |
(2R,3R)-3,5,7-trihydroxy-2-(3-hydroxy-4-methoxyphenyl)-2,3-dihydrochromen-4-one | |
---|---|---|
Source | PubChem | |
URL | https://pubchem.ncbi.nlm.nih.gov | |
Description | Data deposited in or computed by PubChem | |
InChI |
InChI=1S/C16H14O7/c1-22-11-3-2-7(4-9(11)18)16-15(21)14(20)13-10(19)5-8(17)6-12(13)23-16/h2-6,15-19,21H,1H3/t15-,16+/m0/s1 | |
Source | PubChem | |
URL | https://pubchem.ncbi.nlm.nih.gov | |
Description | Data deposited in or computed by PubChem | |
InChI Key |
KQNGHARGJDXHKF-JKSUJKDBSA-N | |
Source | PubChem | |
URL | https://pubchem.ncbi.nlm.nih.gov | |
Description | Data deposited in or computed by PubChem | |
Canonical SMILES |
COC1=C(C=C(C=C1)C2C(C(=O)C3=C(C=C(C=C3O2)O)O)O)O | |
Source | PubChem | |
URL | https://pubchem.ncbi.nlm.nih.gov | |
Description | Data deposited in or computed by PubChem | |
Isomeric SMILES |
COC1=C(C=C(C=C1)[C@@H]2[C@H](C(=O)C3=C(C=C(C=C3O2)O)O)O)O | |
Source | PubChem | |
URL | https://pubchem.ncbi.nlm.nih.gov | |
Description | Data deposited in or computed by PubChem | |
Molecular Formula |
C16H14O7 | |
Source | PubChem | |
URL | https://pubchem.ncbi.nlm.nih.gov | |
Description | Data deposited in or computed by PubChem | |
Molecular Weight |
318.28 g/mol | |
Source | PubChem | |
URL | https://pubchem.ncbi.nlm.nih.gov | |
Description | Data deposited in or computed by PubChem | |
Retrosynthesis Analysis
AI-Powered Synthesis Planning: Our tool employs the Template_relevance Pistachio, Template_relevance Bkms_metabolic, Template_relevance Pistachio_ringbreaker, Template_relevance Reaxys, Template_relevance Reaxys_biocatalysis model, leveraging a vast database of chemical reactions to predict feasible synthetic routes.
One-Step Synthesis Focus: Specifically designed for one-step synthesis, it provides concise and direct routes for your target compounds, streamlining the synthesis process.
Accurate Predictions: Utilizing the extensive PISTACHIO, BKMS_METABOLIC, PISTACHIO_RINGBREAKER, REAXYS, REAXYS_BIOCATALYSIS database, our tool offers high-accuracy predictions, reflecting the latest in chemical research and data.
Strategy Settings
Precursor scoring | Relevance Heuristic |
---|---|
Min. plausibility | 0.01 |
Model | Template_relevance |
Template Set | Pistachio/Bkms_metabolic/Pistachio_ringbreaker/Reaxys/Reaxys_biocatalysis |
Top-N result to add to graph | 6 |
Feasible Synthetic Routes
Descargo de responsabilidad e información sobre productos de investigación in vitro
Tenga en cuenta que todos los artículos e información de productos presentados en BenchChem están destinados únicamente con fines informativos. Los productos disponibles para la compra en BenchChem están diseñados específicamente para estudios in vitro, que se realizan fuera de organismos vivos. Los estudios in vitro, derivados del término latino "in vidrio", involucran experimentos realizados en entornos de laboratorio controlados utilizando células o tejidos. Es importante tener en cuenta que estos productos no se clasifican como medicamentos y no han recibido la aprobación de la FDA para la prevención, tratamiento o cura de ninguna condición médica, dolencia o enfermedad. Debemos enfatizar que cualquier forma de introducción corporal de estos productos en humanos o animales está estrictamente prohibida por ley. Es esencial adherirse a estas pautas para garantizar el cumplimiento de los estándares legales y éticos en la investigación y experimentación.