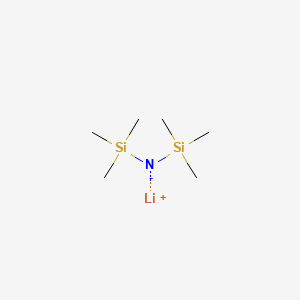
Lithium bis(trimethylsilyl)amide
Descripción general
Descripción
Aplicaciones Científicas De Investigación
As a Strong Non-Nucleophilic Base
LiHMDS serves as a strong non-nucleophilic base in organic chemistry. Its steric hindrance and lower basicity compared to other lithium bases like LDA make it ideal for specific deprotonation reactions. It is particularly useful in the formation of various organolithium compounds, including acetylides and lithium enolates, which are pivotal in carbon-carbon bond-forming reactions such as the Fráter–Seebach alkylation and mixed Claisen condensations .
As a Ligand in Coordination Chemistry
In coordination chemistry, LiHMDS acts as a ligand due to its ability to donate electrons from its nitrogen atom to metal centers. This property is exploited in synthesizing complex metal catalysts that can facilitate a variety of chemical reactions, including polymerization and hydroamination .
In the Synthesis of Heterocyclic Compounds
LiHMDS is employed in the development of new synthetic methodologies for heterocyclic compounds. It is used for the deprotonation of substrates, enabling subsequent cyclization or substitution reactions that lead to the formation of diverse heterocyclic structures .
For the Preparation of Enolates
The compound is instrumental in generating enolates, which are key intermediates in the synthesis of lactone precursors, pyranones, and cyclohexanes. These enolates can undergo further reactions to produce a variety of complex organic molecules .
In Novel Syntheses of Thiadiazoles
LiHMDS is used in a novel three-step synthesis of disubstituted 1,2,5-thiadiazoles. This process involves the use of LiHMDS to initiate reactions that lead to the formation of these sulfur-nitrogen ring compounds, which have significant applications in pharmaceuticals and materials science .
As a Catalyst in Phosphaguanidine Synthesis
The base properties of LiHMDS are harnessed to catalyze the addition of phosphine P-H bonds to carbodiimides, leading to the formation of phosphaguanidines. These compounds have potential applications in various fields, including medicinal chemistry .
In Atomic Layer Deposition (ALD) Processes
LiHMDS serves as a lithium precursor for ALD of textured Li4Ti5O12 as anode material for Li-ion ultrafast charging thin-film batteries. This application underscores its role in advanced material synthesis and energy storage technologies .
As a Scavenger in Electrochemical Applications
In electrochemistry, LiHMDS acts as a scavenger for hydrofluoric acid. It forms an electrochemically robust cathode|electrolyte interphase (CEI) and suppresses side reactions with the electrolyte solution, enhancing the performance and longevity of batteries .
Mecanismo De Acción
Target of Action
Lithium bis(trimethylsilyl)amide, commonly abbreviated as LiHMDS, is a lithiated organosilicon compound . Its primary targets are protons in organic compounds, as it is primarily used as a strong non-nucleophilic base . It is also used as a ligand .
Mode of Action
LiHMDS interacts with its targets by deprotonating them . This interaction results in the formation of various organolithium compounds, including acetylides or lithium enolates . It is relatively more sterically hindered and hence less nucleophilic than other lithium bases .
Biochemical Pathways
LiHMDS is often used in organic chemistry to form various organolithium compounds . It finds use in a range of coupling reactions, particularly carbon-carbon bond-forming reactions such as the Fráter–Seebach alkylation and mixed Claisen condensations . An alternative synthesis of tetrasulfur tetranitride entails the use of S(N(Si(CH3)3)2)2 as a precursor with pre-formed S–N bonds .
Pharmacokinetics
It is worth noting that it is soluble in most nonpolar solvents such as aromatic hydrocarbons, hexanes, and thf .
Result of Action
The result of LiHMDS’s action is the formation of various organolithium compounds, including acetylides or lithium enolates . These compounds are often used in further chemical reactions.
Action Environment
LiHMDS is unstable in air and catches fire when compressed, but it is stable in an atmosphere of nitrogen . It decomposes in water but is soluble in most aprotic solvents . Therefore, the environment significantly influences the action, efficacy, and stability of LiHMDS.
Propiedades
IUPAC Name |
lithium;bis(trimethylsilyl)azanide | |
---|---|---|
Source | PubChem | |
URL | https://pubchem.ncbi.nlm.nih.gov | |
Description | Data deposited in or computed by PubChem | |
InChI |
InChI=1S/C6H18NSi2.Li/c1-8(2,3)7-9(4,5)6;/h1-6H3;/q-1;+1 | |
Source | PubChem | |
URL | https://pubchem.ncbi.nlm.nih.gov | |
Description | Data deposited in or computed by PubChem | |
InChI Key |
YNESATAKKCNGOF-UHFFFAOYSA-N | |
Source | PubChem | |
URL | https://pubchem.ncbi.nlm.nih.gov | |
Description | Data deposited in or computed by PubChem | |
Canonical SMILES |
[Li+].C[Si](C)(C)[N-][Si](C)(C)C | |
Source | PubChem | |
URL | https://pubchem.ncbi.nlm.nih.gov | |
Description | Data deposited in or computed by PubChem | |
Molecular Formula |
C6H18LiNSi2 | |
Source | PubChem | |
URL | https://pubchem.ncbi.nlm.nih.gov | |
Description | Data deposited in or computed by PubChem | |
Related CAS |
999-97-3 (Parent) | |
Record name | Lithium bis(trimethylsilyl)amide | |
Source | ChemIDplus | |
URL | https://pubchem.ncbi.nlm.nih.gov/substance/?source=chemidplus&sourceid=0004039321 | |
Description | ChemIDplus is a free, web search system that provides access to the structure and nomenclature authority files used for the identification of chemical substances cited in National Library of Medicine (NLM) databases, including the TOXNET system. | |
DSSTOX Substance ID |
DTXSID2044426 | |
Record name | Hexamethyldisilazane lithium salt | |
Source | EPA DSSTox | |
URL | https://comptox.epa.gov/dashboard/DTXSID2044426 | |
Description | DSSTox provides a high quality public chemistry resource for supporting improved predictive toxicology. | |
Molecular Weight |
167.4 g/mol | |
Source | PubChem | |
URL | https://pubchem.ncbi.nlm.nih.gov | |
Description | Data deposited in or computed by PubChem | |
Physical Description |
White powder; [Alfa Aesar MSDS] | |
Record name | Lithium bis(trimethylsilyl)amide | |
Source | Haz-Map, Information on Hazardous Chemicals and Occupational Diseases | |
URL | https://haz-map.com/Agents/21119 | |
Description | Haz-Map® is an occupational health database designed for health and safety professionals and for consumers seeking information about the adverse effects of workplace exposures to chemical and biological agents. | |
Explanation | Copyright (c) 2022 Haz-Map(R). All rights reserved. Unless otherwise indicated, all materials from Haz-Map are copyrighted by Haz-Map(R). No part of these materials, either text or image may be used for any purpose other than for personal use. Therefore, reproduction, modification, storage in a retrieval system or retransmission, in any form or by any means, electronic, mechanical or otherwise, for reasons other than personal use, is strictly prohibited without prior written permission. | |
Product Name |
Lithium bis(trimethylsilyl)amide | |
CAS RN |
4039-32-1 | |
Record name | Lithium bis(trimethylsilyl)amide | |
Source | ChemIDplus | |
URL | https://pubchem.ncbi.nlm.nih.gov/substance/?source=chemidplus&sourceid=0004039321 | |
Description | ChemIDplus is a free, web search system that provides access to the structure and nomenclature authority files used for the identification of chemical substances cited in National Library of Medicine (NLM) databases, including the TOXNET system. | |
Record name | Silanamine, 1,1,1-trimethyl-N-(trimethylsilyl)-, lithium salt (1:1) | |
Source | EPA Chemicals under the TSCA | |
URL | https://www.epa.gov/chemicals-under-tsca | |
Description | EPA Chemicals under the Toxic Substances Control Act (TSCA) collection contains information on chemicals and their regulations under TSCA, including non-confidential content from the TSCA Chemical Substance Inventory and Chemical Data Reporting. | |
Record name | Hexamethyldisilazane lithium salt | |
Source | EPA DSSTox | |
URL | https://comptox.epa.gov/dashboard/DTXSID2044426 | |
Description | DSSTox provides a high quality public chemistry resource for supporting improved predictive toxicology. | |
Record name | Lithiumbis(trimethylsilyl)amide | |
Source | European Chemicals Agency (ECHA) | |
URL | https://echa.europa.eu/substance-information/-/substanceinfo/100.021.569 | |
Description | The European Chemicals Agency (ECHA) is an agency of the European Union which is the driving force among regulatory authorities in implementing the EU's groundbreaking chemicals legislation for the benefit of human health and the environment as well as for innovation and competitiveness. | |
Explanation | Use of the information, documents and data from the ECHA website is subject to the terms and conditions of this Legal Notice, and subject to other binding limitations provided for under applicable law, the information, documents and data made available on the ECHA website may be reproduced, distributed and/or used, totally or in part, for non-commercial purposes provided that ECHA is acknowledged as the source: "Source: European Chemicals Agency, http://echa.europa.eu/". Such acknowledgement must be included in each copy of the material. ECHA permits and encourages organisations and individuals to create links to the ECHA website under the following cumulative conditions: Links can only be made to webpages that provide a link to the Legal Notice page. | |
Record name | LITHIUM HEXAMETHYLDISILAZIDE | |
Source | FDA Global Substance Registration System (GSRS) | |
URL | https://gsrs.ncats.nih.gov/ginas/app/beta/substances/RC4N1I108M | |
Description | The FDA Global Substance Registration System (GSRS) enables the efficient and accurate exchange of information on what substances are in regulated products. Instead of relying on names, which vary across regulatory domains, countries, and regions, the GSRS knowledge base makes it possible for substances to be defined by standardized, scientific descriptions. | |
Explanation | Unless otherwise noted, the contents of the FDA website (www.fda.gov), both text and graphics, are not copyrighted. They are in the public domain and may be republished, reprinted and otherwise used freely by anyone without the need to obtain permission from FDA. Credit to the U.S. Food and Drug Administration as the source is appreciated but not required. | |
Synthesis routes and methods I
Procedure details
Synthesis routes and methods II
Procedure details
Synthesis routes and methods III
Procedure details
Synthesis routes and methods IV
Procedure details
Retrosynthesis Analysis
AI-Powered Synthesis Planning: Our tool employs the Template_relevance Pistachio, Template_relevance Bkms_metabolic, Template_relevance Pistachio_ringbreaker, Template_relevance Reaxys, Template_relevance Reaxys_biocatalysis model, leveraging a vast database of chemical reactions to predict feasible synthetic routes.
One-Step Synthesis Focus: Specifically designed for one-step synthesis, it provides concise and direct routes for your target compounds, streamlining the synthesis process.
Accurate Predictions: Utilizing the extensive PISTACHIO, BKMS_METABOLIC, PISTACHIO_RINGBREAKER, REAXYS, REAXYS_BIOCATALYSIS database, our tool offers high-accuracy predictions, reflecting the latest in chemical research and data.
Strategy Settings
Precursor scoring | Relevance Heuristic |
---|---|
Min. plausibility | 0.01 |
Model | Template_relevance |
Template Set | Pistachio/Bkms_metabolic/Pistachio_ringbreaker/Reaxys/Reaxys_biocatalysis |
Top-N result to add to graph | 6 |
Feasible Synthetic Routes
Descargo de responsabilidad e información sobre productos de investigación in vitro
Tenga en cuenta que todos los artículos e información de productos presentados en BenchChem están destinados únicamente con fines informativos. Los productos disponibles para la compra en BenchChem están diseñados específicamente para estudios in vitro, que se realizan fuera de organismos vivos. Los estudios in vitro, derivados del término latino "in vidrio", involucran experimentos realizados en entornos de laboratorio controlados utilizando células o tejidos. Es importante tener en cuenta que estos productos no se clasifican como medicamentos y no han recibido la aprobación de la FDA para la prevención, tratamiento o cura de ninguna condición médica, dolencia o enfermedad. Debemos enfatizar que cualquier forma de introducción corporal de estos productos en humanos o animales está estrictamente prohibida por ley. Es esencial adherirse a estas pautas para garantizar el cumplimiento de los estándares legales y éticos en la investigación y experimentación.