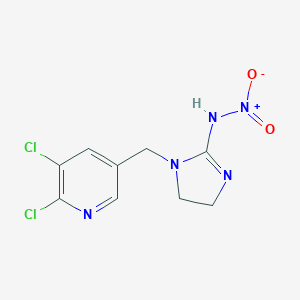
5-Cloro Imidacloprid
Descripción general
Descripción
5-Chloro Imidacloprid: is a derivative of imidacloprid, a systemic insecticide belonging to the neonicotinoid class. This compound is known for its high efficacy in controlling a wide range of insect pests by targeting their central nervous system. It is widely used in agriculture to protect crops from pests such as aphids, whiteflies, and termites .
Aplicaciones Científicas De Investigación
Chemistry: 5-Chloro Imidacloprid is used in the study of neonicotinoid insecticides and their environmental impact. It serves as a model compound for understanding the degradation pathways and persistence of neonicotinoids in the environment .
Biology: In biological research, 5-Chloro Imidacloprid is used to study its effects on non-target organisms, including beneficial insects and soil microbes. It helps in understanding the ecological impact of neonicotinoid insecticides .
Medicine: While not directly used in medicine, the study of 5-Chloro Imidacloprid’s mechanism of action provides insights into the development of new neuroactive compounds that can target specific receptors in the nervous system .
Industry: In the agricultural industry, 5-Chloro Imidacloprid is used as an active ingredient in various insecticidal formulations to protect crops from pest infestations .
Mecanismo De Acción
Target of Action
5-Chloro Imidacloprid, a derivative of Imidacloprid, is a neonicotinoid insecticide . Its primary targets are the nicotinic acetylcholine receptors (nAChRs) in the central nervous system of insects . These receptors play a crucial role in the transmission of nerve impulses .
Mode of Action
5-Chloro Imidacloprid acts by binding to nAChRs, thereby blocking the transmission of nerve impulses . This blockage interferes with the normal neurotransmission, leading to the paralysis and eventual death of the insect . It is effective on contact and via stomach action .
Biochemical Pathways
The biochemical pathways affected by 5-Chloro Imidacloprid primarily involve the disruption of the nicotinergic neuronal pathway . This disruption affects the normal functioning of the insect’s nervous system, leading to its paralysis and death . Imidacloprid forms 5-hydroxy metabolites and olefin metabolites via ethylene hydroxylation and the dehydrogenation pathway .
Pharmacokinetics
Imidacloprid, the parent compound, is known to be rapidly metabolized in mammals . The absorption, distribution, metabolism, and excretion (ADME) properties of 5-Chloro Imidacloprid, and their impact on its bioavailability, are areas of ongoing research .
Result of Action
The result of 5-Chloro Imidacloprid’s action at the molecular and cellular level is the disruption of the insect’s nervous system, leading to paralysis and death . By blocking the transmission of nerve impulses, it prevents the insect from performing normal functions .
Action Environment
5-Chloro Imidacloprid is highly soluble, non-volatile, and persistent in soil . It is moderately mobile and has a low risk of bioaccumulating . It is highly toxic to birds and honeybees, moderately toxic to mammals and earthworms, and non-toxic to fish . Environmental factors such as soil type, temperature, and moisture levels can influence its action, efficacy, and stability .
Análisis Bioquímico
Biochemical Properties
5-Chloro Imidacloprid, like Imidacloprid, is likely to interact with nicotinic acetylcholine receptors in the nervous system of insects . By blocking these receptors, it prevents acetylcholine from transmitting impulses between nerves, leading to the insect’s paralysis and eventual death .
Cellular Effects
Imidacloprid, and by extension 5-Chloro Imidacloprid, has potential cytotoxic and genotoxic effects on human and experimental models . It interferes with the transmission of stimuli in the insect nervous system, causing a blockage of the nicotinergic neuronal pathway . It has been shown to induce reactive oxygen species (ROS) and lysosomal membrane permeabilisation (LMP), causing apoptosis and autophagic dysfunction .
Molecular Mechanism
The molecular mechanism of action of 5-Chloro Imidacloprid is likely similar to that of Imidacloprid. It works by interfering with the transmission of stimuli in the insect nervous system. Specifically, it causes a blockage of the nicotinergic neuronal pathway . By blocking nicotinic acetylcholine receptors, it prevents acetylcholine from transmitting impulses between nerves, resulting in the insect’s paralysis and eventual death .
Temporal Effects in Laboratory Settings
Imidacloprid, the parent compound of 5-Chloro Imidacloprid, has been found to persist in the environment for up to 3000 days . This suggests that 5-Chloro Imidacloprid may also exhibit long-term stability and effects on cellular function in laboratory settings.
Dosage Effects in Animal Models
While specific studies on 5-Chloro Imidacloprid are limited, studies on Imidacloprid have shown that it is rated as “moderately toxic” on an acute oral basis to mammals and low toxicity on a dermal basis . This suggests that the effects of 5-Chloro Imidacloprid may also vary with different dosages in animal models.
Metabolic Pathways
Imidacloprid forms 5-hydroxy metabolites and olefin metabolites via ethylene hydroxylation and the dehydrogenation pathway . Given the structural similarity, 5-Chloro Imidacloprid may be involved in similar metabolic pathways.
Subcellular Localization
As a neonicotinoid, it is known to act on the central nervous system of insects , suggesting that it may localize to nerve cells or synapses.
Métodos De Preparación
Synthetic Routes and Reaction Conditions: The synthesis of 5-Chloro Imidacloprid typically involves the reaction of 2-nitroiminoimidazolidine with 2-chloro-5-chloromethyl pyridine in the presence of an alkali carbonate in an organic solvent. The reaction is carried out under reflux conditions, where the 2-chloro-5-chloromethyl pyridine is gradually added to the mixture .
Industrial Production Methods: In industrial settings, the preparation of 5-Chloro Imidacloprid involves a multi-step process starting from dicyclopentadiene and acraldehyde as initial raw materials. The process includes heating, two-step addition, splitting decomposition, and two-step chlorination to form 2-chlorine-5-nitrapyrin, which then reacts with 2-nitroiminimidazolidine in the presence of catalysts and dehydrating agents .
Análisis De Reacciones Químicas
Types of Reactions: 5-Chloro Imidacloprid undergoes various chemical reactions, including:
Oxidation: The compound can be oxidized to form 5-hydroxy imidacloprid.
Reduction: Reduction reactions can lead to the formation of imidacloprid-guanidine.
Substitution: Substitution reactions can occur at the chlorine atom, leading to different derivatives.
Common Reagents and Conditions:
Oxidation: Permanganate oxidation is commonly used, involving hydroxylation of the C–H bond on the imidazole ring.
Reduction: Common reducing agents include hydrogen gas in the presence of a catalyst.
Substitution: Various nucleophiles can be used to substitute the chlorine atom under mild conditions.
Major Products:
- 5-Hydroxy Imidacloprid
- Imidacloprid-Guanidine
- 6-Chloronicotinic Acid
Comparación Con Compuestos Similares
- Imidacloprid
- Nitenpyram
- Acetamiprid
- Thiamethoxam
- Clothianidin
Comparison: 5-Chloro Imidacloprid is unique due to the presence of a chlorine atom, which enhances its insecticidal activity and environmental persistence. Compared to other neonicotinoids like nitenpyram and acetamiprid, 5-Chloro Imidacloprid has a broader spectrum of activity and is more effective against resistant insect populations .
Propiedades
IUPAC Name |
N-[1-[(5,6-dichloropyridin-3-yl)methyl]-4,5-dihydroimidazol-2-yl]nitramide | |
---|---|---|
Source | PubChem | |
URL | https://pubchem.ncbi.nlm.nih.gov | |
Description | Data deposited in or computed by PubChem | |
InChI |
InChI=1S/C9H9Cl2N5O2/c10-7-3-6(4-13-8(7)11)5-15-2-1-12-9(15)14-16(17)18/h3-4H,1-2,5H2,(H,12,14) | |
Source | PubChem | |
URL | https://pubchem.ncbi.nlm.nih.gov | |
Description | Data deposited in or computed by PubChem | |
InChI Key |
FRLLOFWBBYUDAN-UHFFFAOYSA-N | |
Source | PubChem | |
URL | https://pubchem.ncbi.nlm.nih.gov | |
Description | Data deposited in or computed by PubChem | |
Canonical SMILES |
C1CN(C(=N1)N[N+](=O)[O-])CC2=CC(=C(N=C2)Cl)Cl | |
Source | PubChem | |
URL | https://pubchem.ncbi.nlm.nih.gov | |
Description | Data deposited in or computed by PubChem | |
Molecular Formula |
C9H9Cl2N5O2 | |
Source | PubChem | |
URL | https://pubchem.ncbi.nlm.nih.gov | |
Description | Data deposited in or computed by PubChem | |
Molecular Weight |
290.10 g/mol | |
Source | PubChem | |
URL | https://pubchem.ncbi.nlm.nih.gov | |
Description | Data deposited in or computed by PubChem | |
Retrosynthesis Analysis
AI-Powered Synthesis Planning: Our tool employs the Template_relevance Pistachio, Template_relevance Bkms_metabolic, Template_relevance Pistachio_ringbreaker, Template_relevance Reaxys, Template_relevance Reaxys_biocatalysis model, leveraging a vast database of chemical reactions to predict feasible synthetic routes.
One-Step Synthesis Focus: Specifically designed for one-step synthesis, it provides concise and direct routes for your target compounds, streamlining the synthesis process.
Accurate Predictions: Utilizing the extensive PISTACHIO, BKMS_METABOLIC, PISTACHIO_RINGBREAKER, REAXYS, REAXYS_BIOCATALYSIS database, our tool offers high-accuracy predictions, reflecting the latest in chemical research and data.
Strategy Settings
Precursor scoring | Relevance Heuristic |
---|---|
Min. plausibility | 0.01 |
Model | Template_relevance |
Template Set | Pistachio/Bkms_metabolic/Pistachio_ringbreaker/Reaxys/Reaxys_biocatalysis |
Top-N result to add to graph | 6 |
Feasible Synthetic Routes
Descargo de responsabilidad e información sobre productos de investigación in vitro
Tenga en cuenta que todos los artículos e información de productos presentados en BenchChem están destinados únicamente con fines informativos. Los productos disponibles para la compra en BenchChem están diseñados específicamente para estudios in vitro, que se realizan fuera de organismos vivos. Los estudios in vitro, derivados del término latino "in vidrio", involucran experimentos realizados en entornos de laboratorio controlados utilizando células o tejidos. Es importante tener en cuenta que estos productos no se clasifican como medicamentos y no han recibido la aprobación de la FDA para la prevención, tratamiento o cura de ninguna condición médica, dolencia o enfermedad. Debemos enfatizar que cualquier forma de introducción corporal de estos productos en humanos o animales está estrictamente prohibida por ley. Es esencial adherirse a estas pautas para garantizar el cumplimiento de los estándares legales y éticos en la investigación y experimentación.