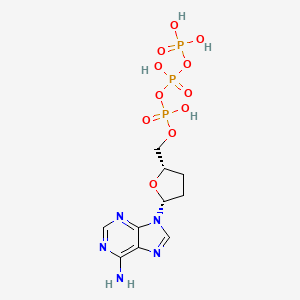
2',3'-Dideoxyadenosine triphosphate
Descripción general
Descripción
2',3'-Dideoxyadenosine triphosphate (dATP) is a naturally occurring nucleotide that is found in all living organisms. It is an essential building block for the synthesis of DNA and RNA molecules. dATP is used in a variety of biochemical and physiological processes, including DNA and RNA synthesis, gene expression, and cell signaling. It is also used in laboratory experiments to study the structure and function of proteins and nucleic acids.
Aplicaciones Científicas De Investigación
Antiviral Drug Synthesis
ddATP is a key component in the synthesis of antiviral drugs, particularly those used to treat HIV. It serves as a precursor for the production of several anti-HIV drugs, including stavudine (d4T), zalcitabine (ddC) , and didanosine (ddI) . These drugs are classified as Nucleoside Reverse Transcriptase Inhibitors (NRTIs) and work by inhibiting the reverse transcriptase enzyme, which is crucial for the replication of HIV.
Chain Termination in DNA Sequencing
In the Sanger method of DNA sequencing, ddATP is used as a chain-terminating nucleotide. Its incorporation into a growing DNA strand prevents further extension due to the absence of a 3’ hydroxyl group, which is necessary for the formation of a phosphodiester bond with the next nucleotide . This property is exploited to generate DNA fragments of varying lengths for sequencing purposes.
Enzyme Mechanistic Studies
ddATP is utilized in mechanistic studies of enzymes involved in nucleic acid metabolism, such as DNA polymerases and reverse transcriptases. By observing the effects of ddATP on these enzymes’ activities, researchers can gain insights into their mechanisms of action and how they interact with nucleotide substrates .
Production of Modified RNA and DNA
Researchers use ddATP to produce RNA and DNA sequences that cannot be extended by polymerases or joined by DNA ligases. This allows for the creation of nucleic acid molecules with specific modifications for experimental and therapeutic applications .
Adenylyl Cyclase Inhibition
ddATP acts as a specific inhibitor of adenylyl cyclase, an enzyme that catalyzes the conversion of ATP to cyclic AMP (cAMP). By modulating cAMP pools within cells, ddATP can be used to study biological processes and pathways that are regulated by cAMP signaling .
Sustainable Synthesis Protocols
Recent research has focused on developing sustainable protocols for synthesizing ddATP and its derivatives. These methods aim to reduce the environmental impact and improve the efficiency of producing ddATP-based compounds, which are crucial for antiviral therapies .
Mecanismo De Acción
Target of Action
The primary target of ddATP is DNA polymerase , an enzyme that plays a crucial role in DNA replication . DNA polymerase is responsible for the synthesis of new DNA strands during replication, and ddATP acts as a chain-elongating inhibitor for this enzyme .
Mode of Action
ddATP interacts with DNA polymerase during the process of DNA replication It is incorporated into the growing DNA strand in place of the regular nucleotide, adenosine triphosphate (ATP). This absence prevents the addition of further nucleotides, thereby terminating the elongation of the DNA chain . This property of ddATP is utilized in the Sanger method of DNA sequencing .
Biochemical Pathways
The incorporation of ddATP into a growing DNA strand disrupts the normal process of DNA replication. When ddATP is incorporated, it terminates the DNA chain, resulting in the production of DNA fragments of varying lengths. These fragments can then be separated and identified, providing the sequence of the original DNA strand . This mechanism is central to the Sanger method of DNA sequencing .
Pharmacokinetics
It’s also known that the compound is active intracellularly .
Result of Action
The primary result of ddATP’s action is the termination of DNA chain elongation during replication. This termination occurs whenever ddATP is incorporated into the growing DNA strand in place of ATP. The resulting DNA fragments can then be used to determine the sequence of the original DNA strand . This property of ddATP has made it a valuable tool in genetic research and biotechnology .
Action Environment
The action of ddATP is influenced by the cellular environment, particularly the presence of DNA polymerase and the nucleotides involved in DNA replication. The efficiency of ddATP incorporation and the subsequent termination of DNA chain elongation can be affected by the concentrations of these molecules. Additionally, factors such as pH and temperature can influence the activity of DNA polymerase and, consequently, the action of ddATP .
Propiedades
IUPAC Name |
[[(2S,5R)-5-(6-aminopurin-9-yl)oxolan-2-yl]methoxy-hydroxyphosphoryl] phosphono hydrogen phosphate | |
---|---|---|
Source | PubChem | |
URL | https://pubchem.ncbi.nlm.nih.gov | |
Description | Data deposited in or computed by PubChem | |
InChI |
InChI=1S/C10H16N5O11P3/c11-9-8-10(13-4-12-9)15(5-14-8)7-2-1-6(24-7)3-23-28(19,20)26-29(21,22)25-27(16,17)18/h4-7H,1-3H2,(H,19,20)(H,21,22)(H2,11,12,13)(H2,16,17,18)/t6-,7+/m0/s1 | |
Source | PubChem | |
URL | https://pubchem.ncbi.nlm.nih.gov | |
Description | Data deposited in or computed by PubChem | |
InChI Key |
OAKPWEUQDVLTCN-NKWVEPMBSA-N | |
Source | PubChem | |
URL | https://pubchem.ncbi.nlm.nih.gov | |
Description | Data deposited in or computed by PubChem | |
Canonical SMILES |
C1CC(OC1COP(=O)(O)OP(=O)(O)OP(=O)(O)O)N2C=NC3=C(N=CN=C32)N | |
Source | PubChem | |
URL | https://pubchem.ncbi.nlm.nih.gov | |
Description | Data deposited in or computed by PubChem | |
Isomeric SMILES |
C1C[C@@H](O[C@@H]1COP(=O)(O)OP(=O)(O)OP(=O)(O)O)N2C=NC3=C(N=CN=C32)N | |
Source | PubChem | |
URL | https://pubchem.ncbi.nlm.nih.gov | |
Description | Data deposited in or computed by PubChem | |
Molecular Formula |
C10H16N5O11P3 | |
Source | PubChem | |
URL | https://pubchem.ncbi.nlm.nih.gov | |
Description | Data deposited in or computed by PubChem | |
DSSTOX Substance ID |
DTXSID90178767 | |
Record name | 2',3'-Dideoxyadenosine triphosphate | |
Source | EPA DSSTox | |
URL | https://comptox.epa.gov/dashboard/DTXSID90178767 | |
Description | DSSTox provides a high quality public chemistry resource for supporting improved predictive toxicology. | |
Molecular Weight |
475.18 g/mol | |
Source | PubChem | |
URL | https://pubchem.ncbi.nlm.nih.gov | |
Description | Data deposited in or computed by PubChem | |
CAS RN |
24027-80-3 | |
Record name | 2',3'-Dideoxyadenosine triphosphate | |
Source | ChemIDplus | |
URL | https://pubchem.ncbi.nlm.nih.gov/substance/?source=chemidplus&sourceid=0024027803 | |
Description | ChemIDplus is a free, web search system that provides access to the structure and nomenclature authority files used for the identification of chemical substances cited in National Library of Medicine (NLM) databases, including the TOXNET system. | |
Record name | 2',3'-Dideoxyadenosine triphosphate | |
Source | DrugBank | |
URL | https://www.drugbank.ca/drugs/DB02189 | |
Description | The DrugBank database is a unique bioinformatics and cheminformatics resource that combines detailed drug (i.e. chemical, pharmacological and pharmaceutical) data with comprehensive drug target (i.e. sequence, structure, and pathway) information. | |
Explanation | Creative Common's Attribution-NonCommercial 4.0 International License (http://creativecommons.org/licenses/by-nc/4.0/legalcode) | |
Record name | 2',3'-Dideoxyadenosine triphosphate | |
Source | EPA DSSTox | |
URL | https://comptox.epa.gov/dashboard/DTXSID90178767 | |
Description | DSSTox provides a high quality public chemistry resource for supporting improved predictive toxicology. | |
Record name | DIDEOXYADENOSINE 5'-TRIPHOSPHATE | |
Source | FDA Global Substance Registration System (GSRS) | |
URL | https://gsrs.ncats.nih.gov/ginas/app/beta/substances/9MCI2H1EJ6 | |
Description | The FDA Global Substance Registration System (GSRS) enables the efficient and accurate exchange of information on what substances are in regulated products. Instead of relying on names, which vary across regulatory domains, countries, and regions, the GSRS knowledge base makes it possible for substances to be defined by standardized, scientific descriptions. | |
Explanation | Unless otherwise noted, the contents of the FDA website (www.fda.gov), both text and graphics, are not copyrighted. They are in the public domain and may be republished, reprinted and otherwise used freely by anyone without the need to obtain permission from FDA. Credit to the U.S. Food and Drug Administration as the source is appreciated but not required. | |
Record name | 2',3'-Dideoxyadenosine-5-triphosphate | |
Source | Human Metabolome Database (HMDB) | |
URL | http://www.hmdb.ca/metabolites/HMDB0060728 | |
Description | The Human Metabolome Database (HMDB) is a freely available electronic database containing detailed information about small molecule metabolites found in the human body. | |
Explanation | HMDB is offered to the public as a freely available resource. Use and re-distribution of the data, in whole or in part, for commercial purposes requires explicit permission of the authors and explicit acknowledgment of the source material (HMDB) and the original publication (see the HMDB citing page). We ask that users who download significant portions of the database cite the HMDB paper in any resulting publications. | |
Retrosynthesis Analysis
AI-Powered Synthesis Planning: Our tool employs the Template_relevance Pistachio, Template_relevance Bkms_metabolic, Template_relevance Pistachio_ringbreaker, Template_relevance Reaxys, Template_relevance Reaxys_biocatalysis model, leveraging a vast database of chemical reactions to predict feasible synthetic routes.
One-Step Synthesis Focus: Specifically designed for one-step synthesis, it provides concise and direct routes for your target compounds, streamlining the synthesis process.
Accurate Predictions: Utilizing the extensive PISTACHIO, BKMS_METABOLIC, PISTACHIO_RINGBREAKER, REAXYS, REAXYS_BIOCATALYSIS database, our tool offers high-accuracy predictions, reflecting the latest in chemical research and data.
Strategy Settings
Precursor scoring | Relevance Heuristic |
---|---|
Min. plausibility | 0.01 |
Model | Template_relevance |
Template Set | Pistachio/Bkms_metabolic/Pistachio_ringbreaker/Reaxys/Reaxys_biocatalysis |
Top-N result to add to graph | 6 |
Feasible Synthetic Routes
Q & A
Q1: How does ddATP exert its antiviral activity against HIV?
A1: ddATP acts as a chain terminator of DNA synthesis. It inhibits HIV replication by acting as a competitive inhibitor of HIV-1 reverse transcriptase (HIV-1 RT), the enzyme responsible for transcribing the viral RNA genome into DNA. [, , , , , , ] Once incorporated into the growing DNA chain by HIV-1 RT, the lack of a 3′-hydroxyl group on ddATP prevents the formation of the next phosphodiester bond, halting further DNA elongation and thus blocking viral replication. [, , , , ]
Q2: What makes ddATP selective for HIV-1 RT over human DNA polymerases?
A2: While ddATP can be incorporated by both viral and human DNA polymerases, HIV-1 RT demonstrates a significantly higher affinity for ddATP compared to human DNA polymerases α, β, and γ. [] This difference in binding affinity contributes to the selective inhibition of HIV-1 replication.
Q3: Does ddATP affect any other cellular processes besides viral reverse transcription?
A3: Yes, ddATP can also inhibit terminal deoxynucleotidyl transferase (TdT), an enzyme primarily found in immature lymphocytes. [] TdT adds nucleotides to the ends of DNA, contributing to antibody diversity. Inhibition of TdT by ddATP can lead to selective toxicity in TdT-positive cells. [, ]
Q4: What is the molecular formula and weight of ddATP?
A4: Unfortunately, the provided scientific articles do not explicitly state the molecular formula and weight of ddATP. Please refer to chemical databases like PubChem or ChemSpider for this information.
Q5: Is there any spectroscopic data available for ddATP?
A5: The provided articles mainly discuss the biological activity and metabolism of ddATP and do not delve into detailed spectroscopic characterization. For spectroscopic data, we recommend consulting specialized databases like the NIST Chemistry WebBook.
Q6: These areas are not extensively covered in the provided research. Where can I find more information about them?
A6: For information on material compatibility and stability, catalytic properties, computational studies, and other specialized applications of ddATP, we suggest exploring dedicated chemical databases, review articles focusing on nucleoside analogs, and publications specifically addressing those aspects.
Q7: How do structural modifications to ddATP affect its activity against HIV-1 RT?
A7: Research shows that even minor structural modifications to ddATP can significantly impact its activity against HIV-1 RT. For instance, the substitution of the 3′-hydroxyl group with a fluorine atom in 2′-β-fluoro-2′,3′-dideoxyadenosine triphosphate (F-ddATP) results in a 20-fold decrease in inhibitory activity against HIV-1 RT compared to ddATP. [] This highlights the importance of the 3′-hydroxyl group for efficient chain termination and emphasizes the sensitivity of HIV-1 RT to subtle structural changes in nucleoside analogs.
Q8: What is the significance of the Q151M mutation in HIV-1 RT?
A8: The Q151M mutation in HIV-1 RT, often accompanied by other mutations like A62V, V75I, F77L, and F116Y (collectively known as the Q151M complex), is a major contributor to multi-nucleoside drug resistance. [] This mutation alters the dNTP binding pocket of HIV-1 RT, decreasing its affinity for ddATP and other nucleoside analogs like ddCTP and AZTTP, ultimately reducing their inhibitory effects. []
Q9: Can this resistance be overcome?
A9: Research suggests that α-boranophosphate nucleotide analogs might offer a way to circumvent the multidrug resistance caused by Q151M and other related mutations. These analogs have shown promising results in restoring the binding affinity and incorporation rate of nucleoside analogs into the viral DNA, thus combating resistance. [, ]
Q10: How is the efficacy of ddATP evaluated in laboratory settings?
A11: The efficacy of ddATP is commonly assessed in vitro using cell-based assays that measure its ability to inhibit HIV replication in cultured cells. [, , ] These assays typically involve infecting susceptible cells with HIV in the presence of varying concentrations of ddATP and measuring viral replication after a specific incubation period. The concentration of ddATP required to inhibit viral replication by 50% (EC50) is a standard measure of its antiviral potency.
Q11: How do the in vivo levels of ddATP correlate with its antiviral activity?
A12: Research indicates a direct correlation between intracellular levels of ddATP and its antiviral activity against HIV. [, ] For instance, one study found that peripheral blood mononuclear cells from HIV-infected patients treated with 2′-β-fluoro-2′,3′-dideoxyadenosine (F-ddA), a prodrug of F-ddATP, had F-ddATP levels ranging from 1.5 to 3.5 pmol/106 cells, which correlated with a reduction in viral load. [] This underscores the importance of maintaining adequate intracellular concentrations of ddATP for effective HIV suppression.
Q12: How does HIV-1 develop resistance to ddATP?
A13: HIV-1, like many viruses, can rapidly mutate, leading to the emergence of drug-resistant strains. Mutations in the HIV-1 RT gene, particularly those within the dNTP binding site, can reduce the binding affinity of ddATP and decrease its incorporation efficiency, ultimately leading to drug resistance. [, , , ]
Q13: Does resistance to one drug translate to resistance to others?
A14: Yes, cross-resistance is a significant concern in HIV treatment. The emergence of mutations conferring resistance to one nucleoside analog, like AZT, can often lead to cross-resistance to other nucleoside analogs, including ddATP. This highlights the need for combination therapy to suppress viral replication effectively and minimize the development of drug resistance. [, , ]
Descargo de responsabilidad e información sobre productos de investigación in vitro
Tenga en cuenta que todos los artículos e información de productos presentados en BenchChem están destinados únicamente con fines informativos. Los productos disponibles para la compra en BenchChem están diseñados específicamente para estudios in vitro, que se realizan fuera de organismos vivos. Los estudios in vitro, derivados del término latino "in vidrio", involucran experimentos realizados en entornos de laboratorio controlados utilizando células o tejidos. Es importante tener en cuenta que estos productos no se clasifican como medicamentos y no han recibido la aprobación de la FDA para la prevención, tratamiento o cura de ninguna condición médica, dolencia o enfermedad. Debemos enfatizar que cualquier forma de introducción corporal de estos productos en humanos o animales está estrictamente prohibida por ley. Es esencial adherirse a estas pautas para garantizar el cumplimiento de los estándares legales y éticos en la investigación y experimentación.