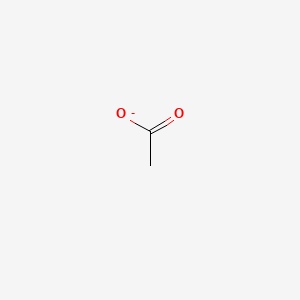
Acetate
Descripción general
Descripción
El acetato es un compuesto químico con la fórmula C₂H₃O₂⁻. Es la base conjugada o ion del ácido acético, que se encuentra normalmente en soluciones acuosas. El acetato es un compuesto versátil que forma sales y ésteres con varios cationes. Se utiliza ampliamente en procesos tanto industriales como biológicos. En la naturaleza, el acetato es un bloque de construcción común para la biosíntesis.
Métodos De Preparación
Rutas Sintéticas y Condiciones de Reacción
El acetato se puede sintetizar mediante varios métodos. Un método común implica la reacción de ácido acético con una base, como el hidróxido de sodio, para formar acetato de sodio y agua:
CH3COOH+NaOH→CH3COONa+H2O
Otro método implica la esterificación del ácido acético con etanol en presencia de un catalizador como el ácido sulfúrico para producir acetato de etilo:
CH3COOH+C2H5OH→CH3COOC2H5+H2O
Métodos de Producción Industrial
Industrialmente, el acetato se produce a gran escala mediante la carbonilación del metanol, que implica la reacción del metanol con monóxido de carbono en presencia de un catalizador para formar ácido acético, que luego se convierte en sales o ésteres de acetato {_svg_1} .
Análisis De Reacciones Químicas
Tipos de Reacciones
El acetato experimenta varias reacciones químicas, que incluyen:
Oxidación: El acetato se puede oxidar a dióxido de carbono y agua.
Reducción: El acetato se puede reducir a etanol.
Sustitución: El acetato puede sufrir reacciones de sustitución nucleófila para formar diferentes ésteres.
Reactivos y Condiciones Comunes
Oxidación: El permanganato de potasio (KMnO₄) o el peróxido de hidrógeno (H₂O₂) se pueden utilizar como agentes oxidantes.
Reducción: El hidruro de litio y aluminio (LiAlH₄) se utiliza comúnmente como agente reductor.
Sustitución: Los cloruros de ácido o los anhídridos se pueden utilizar en presencia de una base para formar ésteres.
Productos Principales
Oxidación: Dióxido de carbono (CO₂) y agua (H₂O).
Reducción: Etanol (C₂H₅OH).
Sustitución: Varios ésteres, como el acetato de etilo (CH₃COOC₂H₅).
Aplicaciones Científicas De Investigación
El acetato tiene numerosas aplicaciones en la investigación científica:
Química: Se utiliza como solvente y reactivo en la síntesis orgánica.
Biología: Desempeña un papel en las vías metabólicas, como el ciclo del ácido cítrico.
Medicina: Se utiliza en la formulación de productos farmacéuticos y como tampón en sistemas biológicos.
Industria: Se utiliza en la producción de polímeros, conservantes de alimentos y como solvente en recubrimientos y tintas .
Mecanismo De Acción
El acetato ejerce sus efectos a través de varios mecanismos:
Vías Metabólicas: El acetato se convierte en acetil-CoA, que ingresa al ciclo del ácido cítrico para producir energía.
Acción de Tampón: El acetato actúa como tampón, manteniendo los niveles de pH en los sistemas biológicos.
Activación Enzimática: El acetato puede activar enzimas involucradas en procesos metabólicos .
Comparación Con Compuestos Similares
Compuestos Similares
Formiato (HCOO⁻): Similar al acetato pero con un átomo de carbono menos.
Propionato (C₃H₅O₂⁻): Similar al acetato pero con un átomo de carbono adicional.
Butirato (C₄H₇O₂⁻): Similar al acetato pero con dos átomos de carbono adicionales.
Singularidad del Acetato
Versatilidad: El acetato puede formar una amplia gama de sales y ésteres.
Importancia Biológica: El acetato es un intermedio clave en las vías metabólicas.
Relevancia Industrial: El acetato se utiliza en varias aplicaciones industriales, desde solventes hasta conservantes de alimentos .
Propiedades
Número CAS |
71-50-1 |
---|---|
Fórmula molecular |
C2H3O2- |
Peso molecular |
59.04 g/mol |
Nombre IUPAC |
acetate |
InChI |
InChI=1S/C2H4O2/c1-2(3)4/h1H3,(H,3,4)/p-1 |
Clave InChI |
QTBSBXVTEAMEQO-UHFFFAOYSA-M |
SMILES |
CC(=O)[O-] |
SMILES canónico |
CC(=O)[O-] |
71-50-1 | |
Origen del producto |
United States |
Synthesis routes and methods I
Procedure details
Synthesis routes and methods II
Procedure details
Synthesis routes and methods III
Procedure details
Synthesis routes and methods IV
Procedure details
Retrosynthesis Analysis
AI-Powered Synthesis Planning: Our tool employs the Template_relevance Pistachio, Template_relevance Bkms_metabolic, Template_relevance Pistachio_ringbreaker, Template_relevance Reaxys, Template_relevance Reaxys_biocatalysis model, leveraging a vast database of chemical reactions to predict feasible synthetic routes.
One-Step Synthesis Focus: Specifically designed for one-step synthesis, it provides concise and direct routes for your target compounds, streamlining the synthesis process.
Accurate Predictions: Utilizing the extensive PISTACHIO, BKMS_METABOLIC, PISTACHIO_RINGBREAKER, REAXYS, REAXYS_BIOCATALYSIS database, our tool offers high-accuracy predictions, reflecting the latest in chemical research and data.
Strategy Settings
Precursor scoring | Relevance Heuristic |
---|---|
Min. plausibility | 0.01 |
Model | Template_relevance |
Template Set | Pistachio/Bkms_metabolic/Pistachio_ringbreaker/Reaxys/Reaxys_biocatalysis |
Top-N result to add to graph | 6 |
Feasible Synthetic Routes
Descargo de responsabilidad e información sobre productos de investigación in vitro
Tenga en cuenta que todos los artículos e información de productos presentados en BenchChem están destinados únicamente con fines informativos. Los productos disponibles para la compra en BenchChem están diseñados específicamente para estudios in vitro, que se realizan fuera de organismos vivos. Los estudios in vitro, derivados del término latino "in vidrio", involucran experimentos realizados en entornos de laboratorio controlados utilizando células o tejidos. Es importante tener en cuenta que estos productos no se clasifican como medicamentos y no han recibido la aprobación de la FDA para la prevención, tratamiento o cura de ninguna condición médica, dolencia o enfermedad. Debemos enfatizar que cualquier forma de introducción corporal de estos productos en humanos o animales está estrictamente prohibida por ley. Es esencial adherirse a estas pautas para garantizar el cumplimiento de los estándares legales y éticos en la investigación y experimentación.