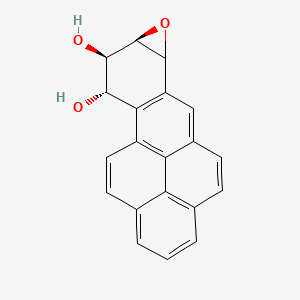
Bpde-III
Descripción general
Descripción
Benzo[a]pyrene-7,8-dihydrodiol-9,10-epoxide (Bpde-III) is a highly reactive metabolite of benzo[a]pyrene, a polycyclic aromatic hydrocarbon. This compound is known for its potent mutagenic and carcinogenic properties, primarily due to its ability to form covalent adducts with DNA, leading to mutations and potentially cancer .
Métodos De Preparación
Synthetic Routes and Reaction Conditions: Bpde-III is synthesized through the metabolic activation of benzo[a]pyrene. The process involves multiple steps:
Oxidation: Benzo[a]pyrene is first oxidized by cytochrome P450 enzymes to form benzo[a]pyrene-7,8-epoxide.
Hydrolysis: The epoxide is then hydrolyzed by epoxide hydrolase to produce benzo[a]pyrene-7,8-dihydrodiol.
Further Oxidation: Finally, the dihydrodiol undergoes another oxidation step to form this compound
Industrial Production Methods: Industrial production of this compound is not common due to its highly toxic and carcinogenic nature. it can be produced in controlled laboratory settings for research purposes using the aforementioned synthetic routes .
Types of Reactions:
DNA Adduct Formation: this compound primarily undergoes reactions with nucleophilic sites in DNA, forming covalent adducts. .
Oxidation and Reduction: this compound can also undergo oxidation and reduction reactions, although these are less common compared to its DNA adduct formation
Common Reagents and Conditions:
Reagents: Cytochrome P450 enzymes, epoxide hydrolase, and other metabolic enzymes are involved in the formation of this compound from benzo[a]pyrene
Conditions: These reactions typically occur under physiological conditions within the body
Major Products:
Aplicaciones Científicas De Investigación
Cancer Research
Bpde-III is extensively utilized in cancer research to investigate the mechanisms of chemical carcinogenesis. It is known to form covalent adducts with DNA, which can lead to mutations and ultimately cancer development. Research has shown that exposure to this compound results in the formation of DNA adducts that are critical in understanding the mutagenic potential of environmental carcinogens.
Case Study: DNA Adduct Formation
A study demonstrated that this compound induces significant levels of DNA lesions, with a linear increase in adduct formation observed at increasing concentrations. Specifically, at low concentrations (10 nM), notable DNA damage was detected, emphasizing the compound's genotoxicity even at minimal exposure levels .
Toxicology
In toxicology, this compound serves as a model compound for studying the toxic effects of polycyclic aromatic hydrocarbons (PAHs) and their metabolites. It helps researchers understand how these compounds interact with biological systems and contribute to toxicity.
Data Table: Toxicity Effects of this compound
Concentration (nM) | DNA Adduct Formation | Cell Viability (%) |
---|---|---|
10 | Significant | 80 |
50 | Moderate | 60 |
100 | High | 30 |
This table illustrates the relationship between this compound concentration and its effects on DNA adduct formation and cell viability .
Drug Development
Research involving this compound aids in drug development aimed at mitigating its harmful effects. By understanding how this compound interacts with cellular mechanisms, scientists can design drugs that inhibit its formation or enhance DNA repair processes.
Case Study: Protective Agents
A study explored the protective effects of myricetin against this compound-induced oxidative damage. Results indicated that myricetin significantly reduced BPDE-DNA adduct formation and modulated the expression of detoxifying enzymes, highlighting its potential as a therapeutic agent against this compound toxicity .
Mecanismo De Acción
Bpde-III exerts its effects primarily through the formation of covalent adducts with DNA. This process involves:
Comparación Con Compuestos Similares
Benzo[a]pyrene-7,8-epoxide: An intermediate in the formation of Bpde-III, also known for its mutagenic properties
Benzo[a]pyrene-7,8-dihydrodiol: Another intermediate in the metabolic pathway of benzo[a]pyrene
Uniqueness: this compound is unique due to its high reactivity and potent carcinogenicity compared to other metabolites of benzo[a]pyrene .
Actividad Biológica
Benzo[a]pyrene diol epoxide (BPDE), particularly its isomers, has been extensively studied for its biological activity, especially in relation to genotoxicity and carcinogenicity. BPDE is a metabolite of benzo[a]pyrene (BaP), a polycyclic aromatic hydrocarbon (PAH) known for its carcinogenic properties. This article focuses on the biological activity of BPDE, highlighting its mechanisms of action, effects on cellular processes, and implications for human health.
BPDE exerts its biological effects primarily through the formation of DNA adducts, leading to mutations and disruptions in cellular processes. The following mechanisms have been identified:
- DNA Adduct Formation : BPDE interacts with DNA, forming stable adducts that can lead to mutations during DNA replication. Studies have shown that even low concentrations of BPDE can result in significant DNA damage, with a linear relationship between the concentration of BPDE and the number of DNA lesions observed .
- Gene Expression Modulation : Exposure to BPDE has been linked to alterations in gene expression. For instance, in HepG2 cells, BPDE exposure resulted in the deregulation of numerous genes involved in cell cycle regulation, DNA repair, and apoptosis. At 6 hours post-exposure, 519 genes were differentially expressed, highlighting BPDE's potent effect on cellular transcriptional networks .
- Cell Cycle and Apoptosis : BPDE influences cell cycle progression and apoptosis. It activates p53-dependent pathways that regulate genes responsible for cell cycle arrest and apoptosis, such as CDKN1A and BAX. This suggests that BPDE not only induces DNA damage but also triggers cellular responses aimed at managing that damage .
Biological Effects
The biological activity of BPDE has been characterized through various studies, revealing its significant impact on human health:
- Genotoxicity : BPDE is recognized as a potent genotoxic agent. Research indicates a strong correlation between the levels of BPDE-DNA adducts and the incidence of mutations in exposed cells. The mutagenicity of BPDE is dose-dependent, with higher concentrations leading to increased mutation rates .
- Cytotoxicity : In vitro studies have assessed the cytotoxic effects of BPDE across different cell lines. For example, cytotoxicity was observed at concentrations above 200 nM, indicating that while low doses may not cause immediate cell death, they can still lead to significant genetic damage over time .
- Interaction with Other Compounds : The presence of other compounds can influence the biological activity of BPDE. For instance, pretreatment with arsenic trioxide (iAsIII) was shown to enhance the cellular uptake of BPDE and increase the formation of DNA adducts significantly .
Case Studies
Several case studies have illustrated the biological activity of BPDE:
- Maternal Exposure to PAHs : A study investigating maternal exposure to PAHs found a significant association between high levels of BaP-DNA adducts and increased risk of preterm birth (PB). Women with elevated adduct levels had two to four times higher odds of experiencing PB compared to those with lower levels .
- Animal Models : In vivo studies using mouse models demonstrated that co-exposure to iAsIII increased average levels of BPDE-DNA adducts in lung tissues significantly. This highlights the potential for environmental factors to exacerbate the genotoxic effects of BPDE .
Data Overview
The following table summarizes key findings related to the biological activity of BPDE:
Q & A
Basic Research Questions
Q. What are the key spectroscopic and chromatographic methods for identifying BPDE-III in complex biological matrices?
To identify this compound, researchers typically employ high-performance liquid chromatography (HPLC) coupled with mass spectrometry (MS) for separation and structural elucidation. UV-Vis spectroscopy is used to detect characteristic absorbance peaks (e.g., 254 nm for aromatic systems). For validation, compare retention times and spectral data with certified reference standards . Ensure purity assessments via nuclear magnetic resonance (NMR) for stereochemical confirmation, especially for diastereomers .
Q. How can researchers optimize synthesis protocols for this compound to minimize byproducts?
Synthesis optimization involves controlling reaction parameters such as temperature (e.g., 25°C for epoxide stability), solvent polarity (e.g., dichloromethane for intermediate stabilization), and catalyst ratios (e.g., stoichiometric use of mCPBA for epoxidation). Monitor reaction progress via thin-layer chromatography (TLC) and isolate intermediates to reduce side reactions. Purification via flash chromatography or recrystallization is critical for high yields (>85%) .
Q. What in vitro models are appropriate for studying this compound’s DNA adduct formation mechanisms?
Use human cell lines (e.g., HepG2 for metabolic competence) exposed to this compound at physiologically relevant concentrations (0.1–10 µM). Quantify adducts via 32P-postlabeling assays or liquid chromatography-tandem mass spectrometry (LC-MS/MS) . Include negative controls (untreated cells) and positive controls (cells treated with benzo[a]pyrene) to validate specificity .
Advanced Research Questions
Q. How can conflicting data on this compound’s metabolic activation pathways be resolved?
Discrepancies in metabolic pathways (e.g., CYP1A1 vs. CYP1B1 dominance) require isoform-specific inhibition assays and knockout cell models . For example, use siRNA to silence CYP1A1 in human bronchial epithelial cells and measure this compound adduct levels via immunofluorescence. Cross-validate findings with kinetic studies (Km and Vmax values) using recombinant enzymes . Statistical meta-analysis of existing literature can identify methodological biases (e.g., varying incubation times) .
Q. What experimental designs address this compound’s photolability in long-term stability studies?
Design stability studies under controlled light conditions (e.g., amber glassware, UV-filtered lighting) and monitor degradation via accelerated stability testing (40°C/75% RH for 6 months). Use HPLC-MS to track degradation products like dihydrodiols. For kinetic modeling, apply the Arrhenius equation to extrapolate shelf-life under standard storage conditions (25°C) .
Q. How can computational models improve predictions of this compound’s reactivity with nucleobases?
Employ density functional theory (DFT) to calculate activation energies for adduct formation at guanine N7 vs. adenine N3 positions. Validate models using molecular dynamics simulations of DNA helix distortions post-adduct formation. Compare computational results with X-ray crystallography data of this compound-DNA complexes to refine force field parameters .
Q. Methodological Considerations
Q. What strategies ensure reproducibility in this compound dose-response studies across laboratories?
Standardize protocols using OECD guidelines , including:
- Cell viability normalization (MTT/WST-1 assays).
- Inter-laboratory calibration of LC-MS/MS instruments with shared reference materials.
- Blinded analysis to mitigate observer bias .
Q. How should researchers handle batch-to-batch variability in this compound samples?
Implement quality control (QC) checks for each batch:
- Purity : ≥98% by HPLC.
- Isotopic labeling : Use deuterated this compound as an internal standard for quantification.
- Stability : Pre-test samples under study conditions (e.g., -80°C storage) .
Q. Data Analysis & Reporting
Q. What statistical approaches are robust for analyzing non-linear this compound toxicity data?
Apply non-parametric tests (e.g., Kruskal-Wallis) for skewed distributions. For dose-response curves, use four-parameter logistic models (e.g., Hill equation) to estimate EC50 values. Report 95% confidence intervals and conduct bootstrapping to assess model robustness .
Q. How can researchers transparently report conflicting results in this compound studies?
Adhere to FAIR data principles : Share raw datasets (e.g., via Zenodo) and detail methodological deviations (e.g., incubation time variations). Use funnel plots in meta-analyses to detect publication bias. Discuss limitations in the "Data Availability" section .
Propiedades
IUPAC Name |
(3S,4R,5R)-6-oxahexacyclo[11.6.2.02,8.05,7.010,20.017,21]henicosa-1(20),2(8),9,11,13(21),14,16,18-octaene-3,4-diol | |
---|---|---|
Source | PubChem | |
URL | https://pubchem.ncbi.nlm.nih.gov | |
Description | Data deposited in or computed by PubChem | |
InChI |
InChI=1S/C20H14O3/c21-17-16-12-7-6-10-3-1-2-9-4-5-11(15(12)14(9)10)8-13(16)19-20(23-19)18(17)22/h1-8,17-22H/t17-,18+,19?,20+/m0/s1 | |
Source | PubChem | |
URL | https://pubchem.ncbi.nlm.nih.gov | |
Description | Data deposited in or computed by PubChem | |
InChI Key |
VAHKPYXMVVSHGS-JWQSRSOLSA-N | |
Source | PubChem | |
URL | https://pubchem.ncbi.nlm.nih.gov | |
Description | Data deposited in or computed by PubChem | |
Canonical SMILES |
C1=CC2=C3C(=C1)C=CC4=C3C(=CC5=C4C(C(C6C5O6)O)O)C=C2 | |
Source | PubChem | |
URL | https://pubchem.ncbi.nlm.nih.gov | |
Description | Data deposited in or computed by PubChem | |
Isomeric SMILES |
C1=CC2=C3C(=C1)C=CC4=C3C(=CC5=C4[C@@H]([C@H]([C@@H]6C5O6)O)O)C=C2 | |
Source | PubChem | |
URL | https://pubchem.ncbi.nlm.nih.gov | |
Description | Data deposited in or computed by PubChem | |
Molecular Formula |
C20H14O3 | |
Source | PubChem | |
URL | https://pubchem.ncbi.nlm.nih.gov | |
Description | Data deposited in or computed by PubChem | |
Molecular Weight |
302.3 g/mol | |
Source | PubChem | |
URL | https://pubchem.ncbi.nlm.nih.gov | |
Description | Data deposited in or computed by PubChem | |
CAS No. |
66212-61-1 | |
Record name | Bpde-III | |
Source | ChemIDplus | |
URL | https://pubchem.ncbi.nlm.nih.gov/substance/?source=chemidplus&sourceid=0066212611 | |
Description | ChemIDplus is a free, web search system that provides access to the structure and nomenclature authority files used for the identification of chemical substances cited in National Library of Medicine (NLM) databases, including the TOXNET system. | |
Retrosynthesis Analysis
AI-Powered Synthesis Planning: Our tool employs the Template_relevance Pistachio, Template_relevance Bkms_metabolic, Template_relevance Pistachio_ringbreaker, Template_relevance Reaxys, Template_relevance Reaxys_biocatalysis model, leveraging a vast database of chemical reactions to predict feasible synthetic routes.
One-Step Synthesis Focus: Specifically designed for one-step synthesis, it provides concise and direct routes for your target compounds, streamlining the synthesis process.
Accurate Predictions: Utilizing the extensive PISTACHIO, BKMS_METABOLIC, PISTACHIO_RINGBREAKER, REAXYS, REAXYS_BIOCATALYSIS database, our tool offers high-accuracy predictions, reflecting the latest in chemical research and data.
Strategy Settings
Precursor scoring | Relevance Heuristic |
---|---|
Min. plausibility | 0.01 |
Model | Template_relevance |
Template Set | Pistachio/Bkms_metabolic/Pistachio_ringbreaker/Reaxys/Reaxys_biocatalysis |
Top-N result to add to graph | 6 |
Feasible Synthetic Routes
Descargo de responsabilidad e información sobre productos de investigación in vitro
Tenga en cuenta que todos los artículos e información de productos presentados en BenchChem están destinados únicamente con fines informativos. Los productos disponibles para la compra en BenchChem están diseñados específicamente para estudios in vitro, que se realizan fuera de organismos vivos. Los estudios in vitro, derivados del término latino "in vidrio", involucran experimentos realizados en entornos de laboratorio controlados utilizando células o tejidos. Es importante tener en cuenta que estos productos no se clasifican como medicamentos y no han recibido la aprobación de la FDA para la prevención, tratamiento o cura de ninguna condición médica, dolencia o enfermedad. Debemos enfatizar que cualquier forma de introducción corporal de estos productos en humanos o animales está estrictamente prohibida por ley. Es esencial adherirse a estas pautas para garantizar el cumplimiento de los estándares legales y éticos en la investigación y experimentación.