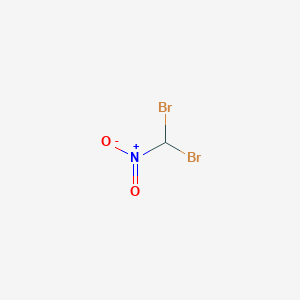
Dibromonitrometileno
Descripción general
Descripción
Dibromonitromethane is an organic compound with the chemical formula CHBr₂NO₂ and a molecular weight of 218.83 g/mol . It is a colorless to pale yellow solid with low solubility in water . This compound is primarily used as a chemical reagent in organic synthesis, particularly in aminomethylation reactions and esterification of carboxylic acids .
Aplicaciones Científicas De Investigación
Dibromonitromethane is widely used in scientific research, particularly in the fields of analytical chemistry and organic synthesis . Its applications include:
Synthesis of Pharmaceuticals: It serves as a reagent in the synthesis of various pharmaceutical compounds.
Agrochemicals: Used in the production of pesticides and herbicides.
Dyes and Pigments: Employed in the synthesis of dyes and pigments for industrial use.
Polymers: Utilized in the creation of polymers and other advanced materials.
Mecanismo De Acción
Dibromonitromethane (DBNM), also known as dibromo(nitro)methane, is an organic compound with the molecular formula CHBr2NO2 . It is a colorless to pale yellow solid and is primarily used as a chemical reagent .
Target of Action
It is often used in organic synthesis reactions, suggesting that its targets could be a wide range of organic compounds .
Mode of Action
It is commonly used in amination reactions and esterification of carboxylic acids , indicating that it may interact with its targets by facilitating the formation of new bonds.
Biochemical Pathways
Given its use in organic synthesis, it is likely involved in various biochemical reactions, depending on the specific context of its use .
Pharmacokinetics
Its solubility in water is relatively low , which could impact its bioavailability and distribution in an aqueous environment.
Result of Action
The molecular and cellular effects of DBNM’s action largely depend on the specific reactions it is used in. As a chemical reagent, it facilitates the formation of new compounds, altering the molecular structure and properties of its targets .
Action Environment
Environmental factors can significantly influence the action, efficacy, and stability of DBNM. For instance, it should be stored at 2-8℃ . Also, it should be handled with appropriate protective equipment to avoid direct contact with skin and eyes, and it should be kept away from flammable and oxidizing substances to prevent fire or explosion .
Métodos De Preparación
Synthetic Routes and Reaction Conditions: Dibromonitromethane can be synthesized through the reaction of bromopicrin with sodium bisulfite at ambient temperature . Another method involves the reaction of 2-bromomethane with silver nitrite at low temperatures, followed by filtration to obtain pure dibromonitromethane .
Industrial Production Methods: Industrial production of dibromonitromethane typically involves the reaction of bromopicrin with sodium bisulfite in an aqueous solution at an acidic pH (between 4.0 and 7.0) . This method is preferred due to its high yield and the avoidance of hazardous organic solvents .
Types of Reactions:
Reduction: It can be reduced to form dibromomethane and other related compounds.
Substitution: Dibromonitromethane can participate in nucleophilic substitution reactions, where the nitro group is replaced by other nucleophiles.
Common Reagents and Conditions:
Oxidation: Common oxidizing agents include potassium permanganate and hydrogen peroxide.
Reduction: Reducing agents such as lithium aluminum hydride and sodium borohydride are commonly used.
Substitution: Nucleophiles like amines and thiols are often employed in substitution reactions.
Major Products:
Oxidation: Brominated organic compounds and nitromethane.
Reduction: Dibromomethane and related halogenated compounds.
Substitution: Various substituted nitromethanes depending on the nucleophile used.
Comparación Con Compuestos Similares
Bromonitromethane (CH₂BrNO₂): Similar in structure but contains only one bromine atom.
Dibromomethane (CH₂Br₂): Lacks the nitro group, making it less reactive in certain types of reactions.
Nitromethane (CH₃NO₂): Contains no bromine atoms, resulting in different reactivity and applications.
Uniqueness: Dibromonitromethane is unique due to its dual functionality, containing both bromine and nitro groups. This dual functionality allows it to participate in a wider range of chemical reactions compared to its similar compounds .
Actividad Biológica
Dibromonitromethane (DBNM) is a halogenated organic compound that has garnered attention due to its biological activity, particularly as a disinfection by-product (DBP). This article delves into the various aspects of its biological activity, including mutagenicity, cytotoxicity, and its role in environmental and health-related contexts.
DBNM is part of a class of compounds known as halonitromethanes, which are formed during the chlorination or bromination of water containing organic matter. These compounds can arise from the reaction of halogens with nitrogen-containing precursors, such as those found in human waste. The formation of DBNM is particularly noted in environments such as swimming pools and drinking water systems where chlorine or bromine is used for disinfection .
Mutagenicity and Cytotoxicity
DBNM has been identified as having mutagenic properties. Studies have shown that it can induce DNA damage in various bacterial strains, including Salmonella species. Specifically, research indicates that DBNM is mutagenic both with and without metabolic activation (S9 mix), suggesting its potential to cause genetic alterations in exposed organisms .
Case Study: Mutagenicity Testing
A comprehensive study assessed the mutagenic effects of several halonitromethanes, including DBNM, using the Salmonella plate-incorporation assay. The results indicated that DBNM exhibited significant mutagenic activity across multiple strains, reinforcing concerns about its potential health risks when present in drinking water or recreational water settings .
Cytotoxic Effects
In addition to its mutagenic properties, DBNM has demonstrated cytotoxic effects on mammalian cells. Research involving Chinese hamster ovary (CHO) cells indicated that exposure to DBNM resulted in dose-dependent cytotoxicity. The data collected showed a significant reduction in cell viability at higher concentrations, highlighting the compound's potential harmful effects on human health .
Summary of Cytotoxicity Data
Concentration (µg/mL) | % Cell Viability |
---|---|
0 | 100 |
10 | 90 |
50 | 70 |
100 | 50 |
200 | 30 |
This table summarizes findings from cytotoxicity assays where increasing concentrations of DBNM led to decreased cell viability, indicating its toxic potential.
Environmental Impact
DBNM's presence in aquatic environments raises concerns about its ecological impact. As a by-product of water treatment processes, it can enter natural water bodies, potentially affecting aquatic life. The compound's stability and persistence in the environment necessitate further studies to understand its long-term effects on ecosystems and human health.
Inhibition of Methanogenesis
Recent studies have explored DBNM's role as an inhibitor of methanogenesis, a critical process in anaerobic digestion and waste treatment. Compounds like DBNM may contribute to reducing methane emissions from agricultural practices by inhibiting methanogenic archaea . This property could be beneficial in mitigating greenhouse gas emissions associated with livestock farming.
Propiedades
IUPAC Name |
dibromo(nitro)methane | |
---|---|---|
Source | PubChem | |
URL | https://pubchem.ncbi.nlm.nih.gov | |
Description | Data deposited in or computed by PubChem | |
InChI |
InChI=1S/CHBr2NO2/c2-1(3)4(5)6/h1H | |
Source | PubChem | |
URL | https://pubchem.ncbi.nlm.nih.gov | |
Description | Data deposited in or computed by PubChem | |
InChI Key |
GQEVYCCMYUNRHJ-UHFFFAOYSA-N | |
Source | PubChem | |
URL | https://pubchem.ncbi.nlm.nih.gov | |
Description | Data deposited in or computed by PubChem | |
Canonical SMILES |
C([N+](=O)[O-])(Br)Br | |
Source | PubChem | |
URL | https://pubchem.ncbi.nlm.nih.gov | |
Description | Data deposited in or computed by PubChem | |
Molecular Formula |
CHBr2NO2 | |
Source | PubChem | |
URL | https://pubchem.ncbi.nlm.nih.gov | |
Description | Data deposited in or computed by PubChem | |
DSSTOX Substance ID |
DTXSID9021637 | |
Record name | Dibromonitromethane | |
Source | EPA DSSTox | |
URL | https://comptox.epa.gov/dashboard/DTXSID9021637 | |
Description | DSSTox provides a high quality public chemistry resource for supporting improved predictive toxicology. | |
Molecular Weight |
218.83 g/mol | |
Source | PubChem | |
URL | https://pubchem.ncbi.nlm.nih.gov | |
Description | Data deposited in or computed by PubChem | |
CAS No. |
598-91-4 | |
Record name | Dibromonitromethane | |
Source | CAS Common Chemistry | |
URL | https://commonchemistry.cas.org/detail?cas_rn=598-91-4 | |
Description | CAS Common Chemistry is an open community resource for accessing chemical information. Nearly 500,000 chemical substances from CAS REGISTRY cover areas of community interest, including common and frequently regulated chemicals, and those relevant to high school and undergraduate chemistry classes. This chemical information, curated by our expert scientists, is provided in alignment with our mission as a division of the American Chemical Society. | |
Explanation | The data from CAS Common Chemistry is provided under a CC-BY-NC 4.0 license, unless otherwise stated. | |
Record name | Methane, dibromonitro- | |
Source | ChemIDplus | |
URL | https://pubchem.ncbi.nlm.nih.gov/substance/?source=chemidplus&sourceid=0000598914 | |
Description | ChemIDplus is a free, web search system that provides access to the structure and nomenclature authority files used for the identification of chemical substances cited in National Library of Medicine (NLM) databases, including the TOXNET system. | |
Record name | Dibromonitromethane | |
Source | EPA DSSTox | |
URL | https://comptox.epa.gov/dashboard/DTXSID9021637 | |
Description | DSSTox provides a high quality public chemistry resource for supporting improved predictive toxicology. | |
Record name | dibromonitromethane | |
Source | European Chemicals Agency (ECHA) | |
URL | https://echa.europa.eu/information-on-chemicals | |
Description | The European Chemicals Agency (ECHA) is an agency of the European Union which is the driving force among regulatory authorities in implementing the EU's groundbreaking chemicals legislation for the benefit of human health and the environment as well as for innovation and competitiveness. | |
Explanation | Use of the information, documents and data from the ECHA website is subject to the terms and conditions of this Legal Notice, and subject to other binding limitations provided for under applicable law, the information, documents and data made available on the ECHA website may be reproduced, distributed and/or used, totally or in part, for non-commercial purposes provided that ECHA is acknowledged as the source: "Source: European Chemicals Agency, http://echa.europa.eu/". Such acknowledgement must be included in each copy of the material. ECHA permits and encourages organisations and individuals to create links to the ECHA website under the following cumulative conditions: Links can only be made to webpages that provide a link to the Legal Notice page. | |
Descargo de responsabilidad e información sobre productos de investigación in vitro
Tenga en cuenta que todos los artículos e información de productos presentados en BenchChem están destinados únicamente con fines informativos. Los productos disponibles para la compra en BenchChem están diseñados específicamente para estudios in vitro, que se realizan fuera de organismos vivos. Los estudios in vitro, derivados del término latino "in vidrio", involucran experimentos realizados en entornos de laboratorio controlados utilizando células o tejidos. Es importante tener en cuenta que estos productos no se clasifican como medicamentos y no han recibido la aprobación de la FDA para la prevención, tratamiento o cura de ninguna condición médica, dolencia o enfermedad. Debemos enfatizar que cualquier forma de introducción corporal de estos productos en humanos o animales está estrictamente prohibida por ley. Es esencial adherirse a estas pautas para garantizar el cumplimiento de los estándares legales y éticos en la investigación y experimentación.