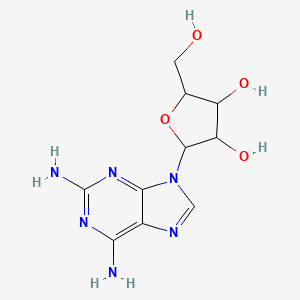
2,6-Diaminopurine riboside
Descripción general
Descripción
2,6-Diaminopurine riboside is a purine nucleoside.
Propiedades
Número CAS |
24649-67-0 |
---|---|
Fórmula molecular |
C10H14N6O4 |
Peso molecular |
282.26 g/mol |
Nombre IUPAC |
2-(2,6-diaminopurin-9-yl)-5-(hydroxymethyl)oxolane-3,4-diol |
InChI |
InChI=1S/C10H14N6O4/c11-7-4-8(15-10(12)14-7)16(2-13-4)9-6(19)5(18)3(1-17)20-9/h2-3,5-6,9,17-19H,1H2,(H4,11,12,14,15) |
Clave InChI |
ZDTFMPXQUSBYRL-UHFFFAOYSA-N |
SMILES |
C1=NC2=C(N=C(N=C2N1C3C(C(C(O3)CO)O)O)N)N |
SMILES canónico |
C1=NC2=C(N=C(N=C2N1C3C(C(C(O3)CO)O)O)N)N |
Key on ui other cas no. |
2096-10-8 |
Sinónimos |
2,6-diaminopurine ribonucleoside 2,6-diaminopurine riboside 2-aminoadenosine |
Origen del producto |
United States |
Synthesis routes and methods I
Procedure details
Synthesis routes and methods II
Procedure details
Retrosynthesis Analysis
AI-Powered Synthesis Planning: Our tool employs the Template_relevance Pistachio, Template_relevance Bkms_metabolic, Template_relevance Pistachio_ringbreaker, Template_relevance Reaxys, Template_relevance Reaxys_biocatalysis model, leveraging a vast database of chemical reactions to predict feasible synthetic routes.
One-Step Synthesis Focus: Specifically designed for one-step synthesis, it provides concise and direct routes for your target compounds, streamlining the synthesis process.
Accurate Predictions: Utilizing the extensive PISTACHIO, BKMS_METABOLIC, PISTACHIO_RINGBREAKER, REAXYS, REAXYS_BIOCATALYSIS database, our tool offers high-accuracy predictions, reflecting the latest in chemical research and data.
Strategy Settings
Precursor scoring | Relevance Heuristic |
---|---|
Min. plausibility | 0.01 |
Model | Template_relevance |
Template Set | Pistachio/Bkms_metabolic/Pistachio_ringbreaker/Reaxys/Reaxys_biocatalysis |
Top-N result to add to graph | 6 |
Feasible Synthetic Routes
Descargo de responsabilidad e información sobre productos de investigación in vitro
Tenga en cuenta que todos los artículos e información de productos presentados en BenchChem están destinados únicamente con fines informativos. Los productos disponibles para la compra en BenchChem están diseñados específicamente para estudios in vitro, que se realizan fuera de organismos vivos. Los estudios in vitro, derivados del término latino "in vidrio", involucran experimentos realizados en entornos de laboratorio controlados utilizando células o tejidos. Es importante tener en cuenta que estos productos no se clasifican como medicamentos y no han recibido la aprobación de la FDA para la prevención, tratamiento o cura de ninguna condición médica, dolencia o enfermedad. Debemos enfatizar que cualquier forma de introducción corporal de estos productos en humanos o animales está estrictamente prohibida por ley. Es esencial adherirse a estas pautas para garantizar el cumplimiento de los estándares legales y éticos en la investigación y experimentación.