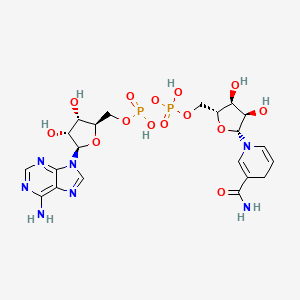
NADH
Descripción general
Descripción
El dinucleótido de nicotinamida y adenina (forma reducida) es una coenzima que se encuentra en todas las células vivas. Desempeña un papel crucial en la respiración celular, donde facilita la conversión de glucosa en energía. El dinucleótido de nicotinamida y adenina (forma reducida) participa en reacciones redox, transportando electrones de una reacción a otra. Existe en dos formas: la forma oxidada (dinucleótido de nicotinamida y adenina) y la forma reducida (dinucleótido de nicotinamida y adenina (forma reducida)) .
Aplicaciones Científicas De Investigación
El dinucleótido de nicotinamida y adenina (forma reducida) tiene numerosas aplicaciones en la investigación científica:
Química: Se utiliza como agente reductor en varias reacciones químicas y como cofactor en ensayos enzimáticos.
Biología: Desempeña un papel vital en el metabolismo celular y la producción de energía.
Mecanismo De Acción
El dinucleótido de nicotinamida y adenina (forma reducida) ejerce sus efectos actuando como un transportador de electrones en reacciones redox. Dona electrones a la cadena de transporte de electrones en las mitocondrias, lo que impulsa la producción de ATP. Los objetivos moleculares del dinucleótido de nicotinamida y adenina (forma reducida) incluyen varias enzimas deshidrogenasas y componentes de la cadena de transporte de electrones .
Análisis Bioquímico
Biochemical Properties
1,4-Dihydronicotinamide adenine dinucleotide is a key molecule in energy metabolism and mitochondrial function. It acts as an electron carrier in redox reactions, transferring electrons from one molecule to another. This process is essential for the production of ATP, the primary energy currency of the cell. NADH interacts with several enzymes, including dehydrogenases, which catalyze the oxidation of substrates by transferring electrons to NAD+, converting it to this compound. Additionally, this compound is involved in non-redox reactions, such as the activation of sirtuins and poly (ADP-ribose) polymerases (PARPs), which play crucial roles in DNA repair, gene expression, and maintaining cellular homeostasis .
Cellular Effects
1,4-Dihydronicotinamide adenine dinucleotide has significant effects on various types of cells and cellular processes. It influences cell function by participating in metabolic pathways that generate ATP, thereby providing the energy required for cellular activities. This compound also impacts cell signaling pathways, such as those involving sirtuins, which regulate gene expression and cellular stress responses. Furthermore, this compound affects cellular metabolism by modulating the activity of enzymes involved in glycolysis, the citric acid cycle, and oxidative phosphorylation .
Molecular Mechanism
The molecular mechanism of 1,4-Dihydronicotinamide adenine dinucleotide involves its role as an electron carrier in redox reactions. This compound donates electrons to the electron transport chain in mitochondria, leading to the production of ATP through oxidative phosphorylation. This process involves the transfer of electrons from this compound to complex I of the electron transport chain, followed by a series of redox reactions that ultimately generate a proton gradient across the mitochondrial membrane. The energy from this gradient is used to synthesize ATP. Additionally, this compound interacts with various enzymes and proteins, influencing their activity and regulating cellular processes such as DNA repair and gene expression .
Temporal Effects in Laboratory Settings
In laboratory settings, the effects of 1,4-Dihydronicotinamide adenine dinucleotide can change over time due to its stability and degradation. This compound is sensitive to environmental factors such as temperature, pH, light, and oxygen. It can be oxidized in the presence of oxygen and hydrolyzed in acidic conditions. Studies have shown that this compound is more stable under anaerobic and alkaline conditions, with lower degradation rates at lower temperatures. Long-term effects of this compound on cellular function have been observed in both in vitro and in vivo studies, indicating its potential for therapeutic applications .
Dosage Effects in Animal Models
The effects of 1,4-Dihydronicotinamide adenine dinucleotide vary with different dosages in animal models. Studies have demonstrated that this compound can enhance NAD+ concentrations in cells, leading to improved energy metabolism and cellular function. High doses of this compound may result in toxic or adverse effects, such as oxidative stress and mitochondrial dysfunction. It is essential to determine the optimal dosage for therapeutic applications to maximize benefits while minimizing potential risks .
Metabolic Pathways
1,4-Dihydronicotinamide adenine dinucleotide is involved in several metabolic pathways, including glycolysis, the citric acid cycle, and oxidative phosphorylation. It acts as an electron carrier, transferring electrons from metabolic intermediates to the electron transport chain, leading to ATP production. This compound also interacts with enzymes such as dehydrogenases, which catalyze the oxidation of substrates and generate this compound from NAD+. These interactions are crucial for maintaining cellular energy balance and metabolic flux .
Transport and Distribution
Within cells and tissues, 1,4-Dihydronicotinamide adenine dinucleotide is transported and distributed through specific transporters and binding proteins. This compound cannot diffuse across membranes, so it relies on transport proteins to move between cellular compartments. In mitochondria, this compound generated in the cytosol is transported into the mitochondrial matrix, where it participates in the electron transport chain. This transport is essential for maintaining cellular energy production and metabolic homeostasis .
Subcellular Localization
1,4-Dihydronicotinamide adenine dinucleotide is localized in various subcellular compartments, including the cytosol and mitochondria. Its activity and function are influenced by its localization, as this compound generated in the cytosol is used for glycolysis, while mitochondrial this compound is involved in oxidative phosphorylation. The subcellular localization of this compound is regulated by targeting signals and post-translational modifications that direct it to specific compartments, ensuring its proper function in cellular metabolism .
Métodos De Preparación
Rutas Sintéticas y Condiciones de Reacción
El dinucleótido de nicotinamida y adenina (forma reducida) puede sintetizarse mediante reacciones enzimáticas que involucran dinucleótido de nicotinamida y adenina e hidrógeno. El proceso generalmente implica el uso de enzimas deshidrogenasas, que catalizan la reducción del dinucleótido de nicotinamida y adenina a dinucleótido de nicotinamida y adenina (forma reducida) en presencia de un donante de hidrógeno adecuado .
Métodos de Producción Industrial
La producción industrial de dinucleótido de nicotinamida y adenina (forma reducida) a menudo involucra procesos de fermentación utilizando microorganismos como la levadura o las bacterias. Estos microorganismos están diseñados para producir en exceso dinucleótido de nicotinamida y adenina (forma reducida) a través de vías metabólicas que involucran la reducción del dinucleótido de nicotinamida y adenina .
Análisis De Reacciones Químicas
Tipos de Reacciones
El dinucleótido de nicotinamida y adenina (forma reducida) principalmente se somete a reacciones redox. Actúa como un agente reductor, donando electrones a otras moléculas. Este proceso es esencial en varias vías metabólicas, incluida la glucólisis, el ciclo del ácido cítrico y la fosforilación oxidativa .
Reactivos y Condiciones Comunes
Los reactivos comunes utilizados en reacciones que involucran dinucleótido de nicotinamida y adenina (forma reducida) incluyen enzimas deshidrogenasas y donantes de hidrógeno. Las reacciones generalmente ocurren en condiciones fisiológicas, como un rango de pH de 7.0 a 8.0 y temperaturas alrededor de 37 °C .
Principales Productos Formados
El principal producto formado por la oxidación del dinucleótido de nicotinamida y adenina (forma reducida) es el dinucleótido de nicotinamida y adenina. Esta reacción es crucial para la producción de trifosfato de adenosina (ATP) en la respiración celular .
Comparación Con Compuestos Similares
El dinucleótido de nicotinamida y adenina (forma reducida) es similar al dinucleótido de nicotinamida y adenina fosfato (forma reducida) en que ambos participan en reacciones redox. El dinucleótido de nicotinamida y adenina fosfato (forma reducida) se utiliza principalmente en reacciones anabólicas, como la síntesis de ácidos grasos y nucleótidos, mientras que el dinucleótido de nicotinamida y adenina (forma reducida) está involucrado principalmente en reacciones catabólicas, como la glucólisis y el ciclo del ácido cítrico .
Lista de Compuestos Similares
- Dinucleótido de nicotinamida y adenina
- Dinucleótido de nicotinamida y adenina fosfato
- Dinucleótido de nicotinamida y adenina fosfato (forma reducida)
El dinucleótido de nicotinamida y adenina (forma reducida) es único en su papel como transportador de electrones central en la respiración celular, lo que lo hace indispensable para la producción de energía en los organismos vivos .
Propiedades
IUPAC Name |
[[(2R,3S,4R,5R)-5-(6-aminopurin-9-yl)-3,4-dihydroxyoxolan-2-yl]methoxy-hydroxyphosphoryl] [(2R,3S,4R,5R)-5-(3-carbamoyl-4H-pyridin-1-yl)-3,4-dihydroxyoxolan-2-yl]methyl hydrogen phosphate | |
---|---|---|
Source | PubChem | |
URL | https://pubchem.ncbi.nlm.nih.gov | |
Description | Data deposited in or computed by PubChem | |
InChI |
InChI=1S/C21H29N7O14P2/c22-17-12-19(25-7-24-17)28(8-26-12)21-16(32)14(30)11(41-21)6-39-44(36,37)42-43(34,35)38-5-10-13(29)15(31)20(40-10)27-3-1-2-9(4-27)18(23)33/h1,3-4,7-8,10-11,13-16,20-21,29-32H,2,5-6H2,(H2,23,33)(H,34,35)(H,36,37)(H2,22,24,25)/t10-,11-,13-,14-,15-,16-,20-,21-/m1/s1 | |
Source | PubChem | |
URL | https://pubchem.ncbi.nlm.nih.gov | |
Description | Data deposited in or computed by PubChem | |
InChI Key |
BOPGDPNILDQYTO-NNYOXOHSSA-N | |
Source | PubChem | |
URL | https://pubchem.ncbi.nlm.nih.gov | |
Description | Data deposited in or computed by PubChem | |
Canonical SMILES |
C1C=CN(C=C1C(=O)N)C2C(C(C(O2)COP(=O)(O)OP(=O)(O)OCC3C(C(C(O3)N4C=NC5=C(N=CN=C54)N)O)O)O)O | |
Source | PubChem | |
URL | https://pubchem.ncbi.nlm.nih.gov | |
Description | Data deposited in or computed by PubChem | |
Isomeric SMILES |
C1C=CN(C=C1C(=O)N)[C@H]2[C@@H]([C@@H]([C@H](O2)COP(=O)(O)OP(=O)(O)OC[C@@H]3[C@H]([C@H]([C@@H](O3)N4C=NC5=C(N=CN=C54)N)O)O)O)O | |
Source | PubChem | |
URL | https://pubchem.ncbi.nlm.nih.gov | |
Description | Data deposited in or computed by PubChem | |
Molecular Formula |
C21H29N7O14P2 | |
Source | PubChem | |
URL | https://pubchem.ncbi.nlm.nih.gov | |
Description | Data deposited in or computed by PubChem | |
DSSTOX Substance ID |
DTXSID30889320 | |
Record name | Adenosine 5'-(trihydrogen diphosphate), P'→5'-ester with 1,4-dihydro-1-.beta.-D-ribofuranosyl-3-pyridinecarboxamide | |
Source | EPA DSSTox | |
URL | https://comptox.epa.gov/dashboard/DTXSID30889320 | |
Description | DSSTox provides a high quality public chemistry resource for supporting improved predictive toxicology. | |
Molecular Weight |
665.4 g/mol | |
Source | PubChem | |
URL | https://pubchem.ncbi.nlm.nih.gov | |
Description | Data deposited in or computed by PubChem | |
Physical Description |
Solid | |
Record name | NADH | |
Source | Human Metabolome Database (HMDB) | |
URL | http://www.hmdb.ca/metabolites/HMDB0001487 | |
Description | The Human Metabolome Database (HMDB) is a freely available electronic database containing detailed information about small molecule metabolites found in the human body. | |
Explanation | HMDB is offered to the public as a freely available resource. Use and re-distribution of the data, in whole or in part, for commercial purposes requires explicit permission of the authors and explicit acknowledgment of the source material (HMDB) and the original publication (see the HMDB citing page). We ask that users who download significant portions of the database cite the HMDB paper in any resulting publications. | |
Mechanism of Action |
NADH is synthesized by the body and thus is not an essential nutrient. It does require the essential nutrient nicotinamide for its synthesis, and its role in energy production is certainly an essential one. In addition to its role in the mitochondrial electron transport chain, NADH is produced in the cytosol. The mitochondrial membrane is impermeable to NADH, and this permeability barrier effectively separates the cytoplasmic from the mitochondrial NADH pools. However, cytoplasmic NADH can be used for biologic energy production. This occurs when the malate-aspartate shuttle introduces reducing equivalents from NADH in the cytosol to the electron transport chain of the mitochondria. This shuttle mainly occurs in the liver and heart. | |
Record name | NADH | |
Source | DrugBank | |
URL | https://www.drugbank.ca/drugs/DB00157 | |
Description | The DrugBank database is a unique bioinformatics and cheminformatics resource that combines detailed drug (i.e. chemical, pharmacological and pharmaceutical) data with comprehensive drug target (i.e. sequence, structure, and pathway) information. | |
Explanation | Creative Common's Attribution-NonCommercial 4.0 International License (http://creativecommons.org/licenses/by-nc/4.0/legalcode) | |
CAS No. |
58-68-4 | |
Record name | NADH | |
Source | CAS Common Chemistry | |
URL | https://commonchemistry.cas.org/detail?cas_rn=58-68-4 | |
Description | CAS Common Chemistry is an open community resource for accessing chemical information. Nearly 500,000 chemical substances from CAS REGISTRY cover areas of community interest, including common and frequently regulated chemicals, and those relevant to high school and undergraduate chemistry classes. This chemical information, curated by our expert scientists, is provided in alignment with our mission as a division of the American Chemical Society. | |
Explanation | The data from CAS Common Chemistry is provided under a CC-BY-NC 4.0 license, unless otherwise stated. | |
Record name | NADH | |
Source | ChemIDplus | |
URL | https://pubchem.ncbi.nlm.nih.gov/substance/?source=chemidplus&sourceid=0000058684 | |
Description | ChemIDplus is a free, web search system that provides access to the structure and nomenclature authority files used for the identification of chemical substances cited in National Library of Medicine (NLM) databases, including the TOXNET system. | |
Record name | NADH | |
Source | DrugBank | |
URL | https://www.drugbank.ca/drugs/DB00157 | |
Description | The DrugBank database is a unique bioinformatics and cheminformatics resource that combines detailed drug (i.e. chemical, pharmacological and pharmaceutical) data with comprehensive drug target (i.e. sequence, structure, and pathway) information. | |
Explanation | Creative Common's Attribution-NonCommercial 4.0 International License (http://creativecommons.org/licenses/by-nc/4.0/legalcode) | |
Record name | Adenosine 5'-(trihydrogen diphosphate), P'.fwdarw.5'-ester with 1,4-dihydro-1-.beta.-D-ribofuranosyl-3-pyridinecarboxamide | |
Source | EPA Chemicals under the TSCA | |
URL | https://www.epa.gov/chemicals-under-tsca | |
Description | EPA Chemicals under the Toxic Substances Control Act (TSCA) collection contains information on chemicals and their regulations under TSCA, including non-confidential content from the TSCA Chemical Substance Inventory and Chemical Data Reporting. | |
Record name | Adenosine 5'-(trihydrogen diphosphate), P'→5'-ester with 1,4-dihydro-1-.beta.-D-ribofuranosyl-3-pyridinecarboxamide | |
Source | EPA DSSTox | |
URL | https://comptox.epa.gov/dashboard/DTXSID30889320 | |
Description | DSSTox provides a high quality public chemistry resource for supporting improved predictive toxicology. | |
Record name | Dihydronicotinamide-adenine dinucleotide | |
Source | European Chemicals Agency (ECHA) | |
URL | https://echa.europa.eu/substance-information/-/substanceinfo/100.000.357 | |
Description | The European Chemicals Agency (ECHA) is an agency of the European Union which is the driving force among regulatory authorities in implementing the EU's groundbreaking chemicals legislation for the benefit of human health and the environment as well as for innovation and competitiveness. | |
Explanation | Use of the information, documents and data from the ECHA website is subject to the terms and conditions of this Legal Notice, and subject to other binding limitations provided for under applicable law, the information, documents and data made available on the ECHA website may be reproduced, distributed and/or used, totally or in part, for non-commercial purposes provided that ECHA is acknowledged as the source: "Source: European Chemicals Agency, http://echa.europa.eu/". Such acknowledgement must be included in each copy of the material. ECHA permits and encourages organisations and individuals to create links to the ECHA website under the following cumulative conditions: Links can only be made to webpages that provide a link to the Legal Notice page. | |
Record name | NADH | |
Source | FDA Global Substance Registration System (GSRS) | |
URL | https://gsrs.ncats.nih.gov/ginas/app/beta/substances/4J24DQ0916 | |
Description | The FDA Global Substance Registration System (GSRS) enables the efficient and accurate exchange of information on what substances are in regulated products. Instead of relying on names, which vary across regulatory domains, countries, and regions, the GSRS knowledge base makes it possible for substances to be defined by standardized, scientific descriptions. | |
Explanation | Unless otherwise noted, the contents of the FDA website (www.fda.gov), both text and graphics, are not copyrighted. They are in the public domain and may be republished, reprinted and otherwise used freely by anyone without the need to obtain permission from FDA. Credit to the U.S. Food and Drug Administration as the source is appreciated but not required. | |
Record name | NADH | |
Source | Human Metabolome Database (HMDB) | |
URL | http://www.hmdb.ca/metabolites/HMDB0001487 | |
Description | The Human Metabolome Database (HMDB) is a freely available electronic database containing detailed information about small molecule metabolites found in the human body. | |
Explanation | HMDB is offered to the public as a freely available resource. Use and re-distribution of the data, in whole or in part, for commercial purposes requires explicit permission of the authors and explicit acknowledgment of the source material (HMDB) and the original publication (see the HMDB citing page). We ask that users who download significant portions of the database cite the HMDB paper in any resulting publications. | |
Melting Point |
140.0-142.0 °C, 140.0 - 142.0 °C | |
Record name | NADH | |
Source | DrugBank | |
URL | https://www.drugbank.ca/drugs/DB00157 | |
Description | The DrugBank database is a unique bioinformatics and cheminformatics resource that combines detailed drug (i.e. chemical, pharmacological and pharmaceutical) data with comprehensive drug target (i.e. sequence, structure, and pathway) information. | |
Explanation | Creative Common's Attribution-NonCommercial 4.0 International License (http://creativecommons.org/licenses/by-nc/4.0/legalcode) | |
Record name | NADH | |
Source | Human Metabolome Database (HMDB) | |
URL | http://www.hmdb.ca/metabolites/HMDB0001487 | |
Description | The Human Metabolome Database (HMDB) is a freely available electronic database containing detailed information about small molecule metabolites found in the human body. | |
Explanation | HMDB is offered to the public as a freely available resource. Use and re-distribution of the data, in whole or in part, for commercial purposes requires explicit permission of the authors and explicit acknowledgment of the source material (HMDB) and the original publication (see the HMDB citing page). We ask that users who download significant portions of the database cite the HMDB paper in any resulting publications. | |
Retrosynthesis Analysis
AI-Powered Synthesis Planning: Our tool employs the Template_relevance Pistachio, Template_relevance Bkms_metabolic, Template_relevance Pistachio_ringbreaker, Template_relevance Reaxys, Template_relevance Reaxys_biocatalysis model, leveraging a vast database of chemical reactions to predict feasible synthetic routes.
One-Step Synthesis Focus: Specifically designed for one-step synthesis, it provides concise and direct routes for your target compounds, streamlining the synthesis process.
Accurate Predictions: Utilizing the extensive PISTACHIO, BKMS_METABOLIC, PISTACHIO_RINGBREAKER, REAXYS, REAXYS_BIOCATALYSIS database, our tool offers high-accuracy predictions, reflecting the latest in chemical research and data.
Strategy Settings
Precursor scoring | Relevance Heuristic |
---|---|
Min. plausibility | 0.01 |
Model | Template_relevance |
Template Set | Pistachio/Bkms_metabolic/Pistachio_ringbreaker/Reaxys/Reaxys_biocatalysis |
Top-N result to add to graph | 6 |
Feasible Synthetic Routes
Descargo de responsabilidad e información sobre productos de investigación in vitro
Tenga en cuenta que todos los artículos e información de productos presentados en BenchChem están destinados únicamente con fines informativos. Los productos disponibles para la compra en BenchChem están diseñados específicamente para estudios in vitro, que se realizan fuera de organismos vivos. Los estudios in vitro, derivados del término latino "in vidrio", involucran experimentos realizados en entornos de laboratorio controlados utilizando células o tejidos. Es importante tener en cuenta que estos productos no se clasifican como medicamentos y no han recibido la aprobación de la FDA para la prevención, tratamiento o cura de ninguna condición médica, dolencia o enfermedad. Debemos enfatizar que cualquier forma de introducción corporal de estos productos en humanos o animales está estrictamente prohibida por ley. Es esencial adherirse a estas pautas para garantizar el cumplimiento de los estándares legales y éticos en la investigación y experimentación.