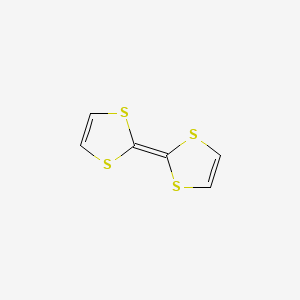
Tetrathiafulvaleno
Descripción general
Descripción
Tetrathiafulvalene (TTF) is an electron-donor which consists of oligomers, dendrimers and polymers which can be used in the formation of redox macromolecules.
Tetrathiafulvalene is an organosulfur heterocyclic compound and a member of fulvalenes.
Aplicaciones Científicas De Investigación
Metales Orgánicos
TTF es una de las moléculas más versátiles y conocidas que exhibe propiedades redox excepcionales y un notable carácter donador de electrones {svg_1}. Tiene una importancia significativa en el desarrollo de materiales conductores de electricidad, también conocidos como metales orgánicos o sintéticos {svg_2}.
Electrónica Molecular
TTF ha contribuido al desarrollo de la electrónica molecular {svg_3} {svg_4}. Sus propiedades únicas lo convierten en un candidato adecuado para la construcción de dispositivos electrónicos a nivel molecular {svg_5}.
Sistemas Supramoleculares
TTF ha sido ampliamente explorado como un donador de electrones π en sistemas supramoleculares {svg_6}. Se ha utilizado en la construcción de arquitecturas elaboradas basadas en TTF y aprovechando los sistemas resultantes en el contexto del reconocimiento supramolecular huésped-huésped {svg_7}.
Materiales Optoelectrónicos
El carácter inherente de donación de electrones de los derivados de TTF ha llevado a su uso en la construcción de materiales optoelectrónicos altamente eficientes {svg_8}. Estos materiales tienen aplicaciones en dispositivos como células solares, diodos emisores de luz (LED) y fotodetectores.
Sensores Ópticos
Los sistemas basados en TTF se han utilizado en el desarrollo de sensores ópticos {svg_9}. Estos sensores pueden detectar cambios en su entorno basados en cambios en sus propiedades ópticas.
Conjuntos de Transferencia de Electrones
TTF se utiliza en la construcción de conjuntos de transferencia de electrones {svg_10}. Estos son sistemas donde la transferencia de electrones ocurre entre dos o más componentes, y tienen aplicaciones en áreas como el almacenamiento y conversión de energía.
Superconductores Orgánicos
TTF se utiliza en el desarrollo de superconductores orgánicos {svg_11} {svg_12}. Estos son materiales que pueden conducir electricidad sin resistencia a bajas temperaturas.
Máquinas Moleculares
Los sistemas basados en TTF se han utilizado en la construcción de "máquinas moleculares" {svg_13}. Estos son sistemas que pueden realizar trabajo mecánico a nivel molecular, y tienen aplicaciones potenciales en nanotecnología {svg_14}.
Mecanismo De Acción
Target of Action
Tetrathiafulvalene (TTF) is a versatile molecule known for its outstanding redox properties and remarkable electron donor character . It primarily targets the development of electrically conducting materials, also known as organic or synthetic metals . It also plays a significant role in molecular electronics .
Mode of Action
TTF exhibits a strong interaction with its targets due to its inherent electron-donating character . It can be reversibly oxidized twice, which contributes to its high electrical conductivity . The planarity of TTF allows for π-π stacking of its oxidized derivatives, its high symmetry promotes charge delocalization, thereby minimizing coulombic repulsions .
Biochemical Pathways
TTF has been extensively explored as a π-electron donor in supramolecular systems . Over the last two decades, substantial advances have been made in terms of constructing elaborate architectures based on TTF and exploiting the resulting systems in the context of supramolecular host–guest recognition . The inherent electron-donating character of TTF derivatives has led to their use in the construction of highly efficient optoelectronic materials, optical sensors, and electron-transfer ensembles .
Pharmacokinetics
It’s known that ttf is insoluble in water but soluble in organic solvents .
Result of Action
The result of TTF’s action is the development of materials with high electrical conductivity . Its oxidized derivatives, such as salts derived from TTF+, exhibit distinctive electrical properties . Furthermore, TTF-based covalent organic frameworks (COFs) have been reported to markedly improve both iodine adsorption capacity and adsorption kinetics due to their strong interaction .
Action Environment
The action of TTF can be influenced by environmental factors. For instance, TTF-based COFs have been used for iodine capture, demonstrating the synergistic effect of physical and chemical adsorption of iodine . This shows that the action, efficacy, and stability of TTF can be influenced by the presence of certain elements in the environment .
Análisis Bioquímico
Biochemical Properties
Tetrathiafulvalene plays a significant role in biochemical reactions due to its outstanding redox properties. It acts as an electron donor and interacts with various biomolecules, including enzymes and proteins. The compound’s ability to undergo reversible oxidation makes it a valuable tool in studying redox reactions and electron transfer processes. Tetrathiafulvalene interacts with enzymes such as oxidoreductases, which facilitate electron transfer by accepting or donating electrons . These interactions are crucial in understanding the mechanisms of redox reactions in biological systems.
Cellular Effects
Tetrathiafulvalene influences various cellular processes, including cell signaling pathways, gene expression, and cellular metabolism. The compound’s redox properties enable it to modulate the activity of redox-sensitive signaling molecules, thereby affecting cell function. For instance, Tetrathiafulvalene can alter the redox state of transcription factors, leading to changes in gene expression . Additionally, the compound’s interaction with cellular enzymes can impact metabolic pathways, influencing cellular energy production and overall metabolism.
Molecular Mechanism
At the molecular level, Tetrathiafulvalene exerts its effects through redox interactions with biomolecules. The compound can be oxidized to form stable radical cations, which can further interact with other molecules. These redox interactions can lead to the activation or inhibition of enzymes, depending on the specific biochemical context . For example, Tetrathiafulvalene can inhibit certain oxidoreductases by competing with natural substrates for electron transfer, thereby modulating the enzyme’s activity.
Temporal Effects in Laboratory Settings
In laboratory settings, the effects of Tetrathiafulvalene can change over time due to its stability and degradation properties. The compound is relatively stable under controlled conditions but can degrade when exposed to air or light. Long-term studies have shown that Tetrathiafulvalene can have sustained effects on cellular function, particularly in redox-sensitive pathways . Its stability and activity can diminish over time, necessitating careful handling and storage in experimental setups.
Dosage Effects in Animal Models
The effects of Tetrathiafulvalene vary with different dosages in animal models. At low doses, the compound can enhance redox signaling and improve cellular function. At high doses, Tetrathiafulvalene can exhibit toxic effects, including oxidative stress and cellular damage . Studies have shown that there is a threshold beyond which the compound’s beneficial effects are outweighed by its toxicity, highlighting the importance of dosage optimization in experimental and therapeutic applications.
Metabolic Pathways
Tetrathiafulvalene is involved in various metabolic pathways, primarily those related to redox reactions. The compound interacts with enzymes such as oxidoreductases and cofactors like NADH and FADH2, which are essential for electron transfer processes . These interactions can influence metabolic flux and alter the levels of key metabolites, impacting overall cellular metabolism.
Transport and Distribution
Within cells and tissues, Tetrathiafulvalene is transported and distributed through interactions with transporters and binding proteins. The compound’s lipophilic nature allows it to diffuse across cell membranes, while specific transporters facilitate its movement within the cell . Tetrathiafulvalene can accumulate in certain cellular compartments, influencing its localization and activity.
Subcellular Localization
Tetrathiafulvalene’s subcellular localization is influenced by targeting signals and post-translational modifications. The compound can be directed to specific compartments or organelles, such as mitochondria, where it can exert its redox effects
Actividad Biológica
Tetrathiafulvalene (TTF) is a unique organic compound known for its remarkable redox properties and potential applications in various biological systems. This article explores the biological activity of TTF, focusing on its synthesis, mechanisms of action, and implications for therapeutic applications.
Overview of Tetrathiafulvalene
Tetrathiafulvalene is characterized by its planar structure and ability to act as a p-type electron donor. Its redox properties allow it to participate in electron transfer processes, making it a candidate for various biological applications, including drug design and molecular sensing.
Synthesis of Tetrathiafulvalene Derivatives
Recent studies have focused on synthesizing TTF derivatives that enhance its biological activity. For instance, water-soluble TTF derivatives containing carbohydrate moieties have been synthesized to explore their interaction with biological systems. These derivatives are hypothesized to participate in metabolic pathways influenced by redox reactions, potentially affecting cellular processes such as membrane transport and DNA intercalation .
Anticancer Activity
TTF and its derivatives have been evaluated for their anticancer properties. In vitro assays using human neuroblastoma cell lines (SK-N-LO and SK-N-SH) demonstrated that specific TTF derivatives exhibited significant cytotoxic effects. The MTT assay results indicated that certain derivatives could induce cell death at concentrations lower than 100 μM, suggesting their potential as anticancer agents .
Table 1: Cytotoxicity of TTF Derivatives on Neuroblastoma Cell Lines
Compound | IC50 (μM) | Cell Line |
---|---|---|
TTF-Glucose | 45 ± 5 | SK-N-LO |
TTF-Galactose | 60 ± 7 | SK-N-SH |
TTF-Modified A | 30 ± 3 | SK-N-LO |
TTF-Modified B | 80 ± 10 | SK-N-SH |
Antibacterial Activity
TTF has also been linked to antibacterial properties. A study synthesized cyclic sulfamide linked to TTF and evaluated its antibacterial activity against various bacterial strains. The results indicated that these compounds exhibited significant antibacterial effects, with minimum inhibitory concentrations (MICs) comparable to standard antibiotics .
Table 2: Antibacterial Activity of TTF Derivatives
Compound | MIC (μg/mL) | Bacterial Strain |
---|---|---|
Cyclic Sulfamide-TTF | 25 | E. coli |
Cyclic Sulfamide-TTF | 30 | S. aureus |
Cyclic Sulfamide-TTF | 20 | P. aeruginosa |
The mechanisms underlying the biological activity of TTF are multifaceted:
- Redox Activity : The ability of TTF to undergo reversible oxidation and reduction reactions allows it to modulate cellular redox states, which can influence signaling pathways related to cell proliferation and apoptosis.
- DNA Interaction : Due to its planar structure, TTF can intercalate into DNA, potentially disrupting replication and transcription processes in cancer cells.
- Membrane Transport Modulation : The incorporation of carbohydrate moieties enhances the solubility of TTF derivatives, facilitating their transport across cellular membranes, which is crucial for their bioactivity .
Case Studies
- Neuroblastoma Treatment : A study investigated the effects of a specific TTF derivative on neuroblastoma cells, revealing that it could induce apoptosis through oxidative stress mechanisms. This highlights the potential use of TTF derivatives in targeted cancer therapies.
- Antimicrobial Applications : Research into cyclic sulfamide-TFF derivatives demonstrated promising results against resistant bacterial strains, suggesting that these compounds could serve as novel antimicrobial agents in the face of rising antibiotic resistance .
Propiedades
IUPAC Name |
2-(1,3-dithiol-2-ylidene)-1,3-dithiole | |
---|---|---|
Source | PubChem | |
URL | https://pubchem.ncbi.nlm.nih.gov | |
Description | Data deposited in or computed by PubChem | |
InChI |
InChI=1S/C6H4S4/c1-2-8-5(7-1)6-9-3-4-10-6/h1-4H | |
Source | PubChem | |
URL | https://pubchem.ncbi.nlm.nih.gov | |
Description | Data deposited in or computed by PubChem | |
InChI Key |
FHCPAXDKURNIOZ-UHFFFAOYSA-N | |
Source | PubChem | |
URL | https://pubchem.ncbi.nlm.nih.gov | |
Description | Data deposited in or computed by PubChem | |
Canonical SMILES |
C1=CSC(=C2SC=CS2)S1 | |
Source | PubChem | |
URL | https://pubchem.ncbi.nlm.nih.gov | |
Description | Data deposited in or computed by PubChem | |
Molecular Formula |
C6H4S4 | |
Source | PubChem | |
URL | https://pubchem.ncbi.nlm.nih.gov | |
Description | Data deposited in or computed by PubChem | |
Related CAS |
56388-66-0 | |
Record name | 1,3-Dithiole, 2-(1,3-dithiol-2-ylidene)-, homopolymer | |
Source | CAS Common Chemistry | |
URL | https://commonchemistry.cas.org/detail?cas_rn=56388-66-0 | |
Description | CAS Common Chemistry is an open community resource for accessing chemical information. Nearly 500,000 chemical substances from CAS REGISTRY cover areas of community interest, including common and frequently regulated chemicals, and those relevant to high school and undergraduate chemistry classes. This chemical information, curated by our expert scientists, is provided in alignment with our mission as a division of the American Chemical Society. | |
Explanation | The data from CAS Common Chemistry is provided under a CC-BY-NC 4.0 license, unless otherwise stated. | |
DSSTOX Substance ID |
DTXSID6067620 | |
Record name | 1,3-Dithiole, 2-(1,3-dithiol-2-ylidene)- | |
Source | EPA DSSTox | |
URL | https://comptox.epa.gov/dashboard/DTXSID6067620 | |
Description | DSSTox provides a high quality public chemistry resource for supporting improved predictive toxicology. | |
Molecular Weight |
204.4 g/mol | |
Source | PubChem | |
URL | https://pubchem.ncbi.nlm.nih.gov | |
Description | Data deposited in or computed by PubChem | |
Physical Description |
Orange or yellow solid in neutral state; Loses electrons forming purple cation and yellow dication; [Merck Index] Orange crystalline powder; [Alfa Aesar MSDS] | |
Record name | Tetrathiafulvalene | |
Source | Haz-Map, Information on Hazardous Chemicals and Occupational Diseases | |
URL | https://haz-map.com/Agents/19865 | |
Description | Haz-Map® is an occupational health database designed for health and safety professionals and for consumers seeking information about the adverse effects of workplace exposures to chemical and biological agents. | |
Explanation | Copyright (c) 2022 Haz-Map(R). All rights reserved. Unless otherwise indicated, all materials from Haz-Map are copyrighted by Haz-Map(R). No part of these materials, either text or image may be used for any purpose other than for personal use. Therefore, reproduction, modification, storage in a retrieval system or retransmission, in any form or by any means, electronic, mechanical or otherwise, for reasons other than personal use, is strictly prohibited without prior written permission. | |
CAS No. |
31366-25-3 | |
Record name | Tetrathiafulvalene | |
Source | CAS Common Chemistry | |
URL | https://commonchemistry.cas.org/detail?cas_rn=31366-25-3 | |
Description | CAS Common Chemistry is an open community resource for accessing chemical information. Nearly 500,000 chemical substances from CAS REGISTRY cover areas of community interest, including common and frequently regulated chemicals, and those relevant to high school and undergraduate chemistry classes. This chemical information, curated by our expert scientists, is provided in alignment with our mission as a division of the American Chemical Society. | |
Explanation | The data from CAS Common Chemistry is provided under a CC-BY-NC 4.0 license, unless otherwise stated. | |
Record name | Tetrathiafulvalene | |
Source | ChemIDplus | |
URL | https://pubchem.ncbi.nlm.nih.gov/substance/?source=chemidplus&sourceid=0031366253 | |
Description | ChemIDplus is a free, web search system that provides access to the structure and nomenclature authority files used for the identification of chemical substances cited in National Library of Medicine (NLM) databases, including the TOXNET system. | |
Record name | 31366-25-3 | |
Source | DTP/NCI | |
URL | https://dtp.cancer.gov/dtpstandard/servlet/dwindex?searchtype=NSC&outputformat=html&searchlist=222862 | |
Description | The NCI Development Therapeutics Program (DTP) provides services and resources to the academic and private-sector research communities worldwide to facilitate the discovery and development of new cancer therapeutic agents. | |
Explanation | Unless otherwise indicated, all text within NCI products is free of copyright and may be reused without our permission. Credit the National Cancer Institute as the source. | |
Record name | 1,3-Dithiole, 2-(1,3-dithiol-2-ylidene)- | |
Source | EPA Chemicals under the TSCA | |
URL | https://www.epa.gov/chemicals-under-tsca | |
Description | EPA Chemicals under the Toxic Substances Control Act (TSCA) collection contains information on chemicals and their regulations under TSCA, including non-confidential content from the TSCA Chemical Substance Inventory and Chemical Data Reporting. | |
Record name | 1,3-Dithiole, 2-(1,3-dithiol-2-ylidene)- | |
Source | EPA DSSTox | |
URL | https://comptox.epa.gov/dashboard/DTXSID6067620 | |
Description | DSSTox provides a high quality public chemistry resource for supporting improved predictive toxicology. | |
Record name | δ-2:2'-bis(1,3-dithiazole) | |
Source | European Chemicals Agency (ECHA) | |
URL | https://echa.europa.eu/substance-information/-/substanceinfo/100.045.979 | |
Description | The European Chemicals Agency (ECHA) is an agency of the European Union which is the driving force among regulatory authorities in implementing the EU's groundbreaking chemicals legislation for the benefit of human health and the environment as well as for innovation and competitiveness. | |
Explanation | Use of the information, documents and data from the ECHA website is subject to the terms and conditions of this Legal Notice, and subject to other binding limitations provided for under applicable law, the information, documents and data made available on the ECHA website may be reproduced, distributed and/or used, totally or in part, for non-commercial purposes provided that ECHA is acknowledged as the source: "Source: European Chemicals Agency, http://echa.europa.eu/". Such acknowledgement must be included in each copy of the material. ECHA permits and encourages organisations and individuals to create links to the ECHA website under the following cumulative conditions: Links can only be made to webpages that provide a link to the Legal Notice page. | |
Record name | TETRATHIAFULVALENE | |
Source | FDA Global Substance Registration System (GSRS) | |
URL | https://gsrs.ncats.nih.gov/ginas/app/beta/substances/HY1EN16W9T | |
Description | The FDA Global Substance Registration System (GSRS) enables the efficient and accurate exchange of information on what substances are in regulated products. Instead of relying on names, which vary across regulatory domains, countries, and regions, the GSRS knowledge base makes it possible for substances to be defined by standardized, scientific descriptions. | |
Explanation | Unless otherwise noted, the contents of the FDA website (www.fda.gov), both text and graphics, are not copyrighted. They are in the public domain and may be republished, reprinted and otherwise used freely by anyone without the need to obtain permission from FDA. Credit to the U.S. Food and Drug Administration as the source is appreciated but not required. | |
Retrosynthesis Analysis
AI-Powered Synthesis Planning: Our tool employs the Template_relevance Pistachio, Template_relevance Bkms_metabolic, Template_relevance Pistachio_ringbreaker, Template_relevance Reaxys, Template_relevance Reaxys_biocatalysis model, leveraging a vast database of chemical reactions to predict feasible synthetic routes.
One-Step Synthesis Focus: Specifically designed for one-step synthesis, it provides concise and direct routes for your target compounds, streamlining the synthesis process.
Accurate Predictions: Utilizing the extensive PISTACHIO, BKMS_METABOLIC, PISTACHIO_RINGBREAKER, REAXYS, REAXYS_BIOCATALYSIS database, our tool offers high-accuracy predictions, reflecting the latest in chemical research and data.
Strategy Settings
Precursor scoring | Relevance Heuristic |
---|---|
Min. plausibility | 0.01 |
Model | Template_relevance |
Template Set | Pistachio/Bkms_metabolic/Pistachio_ringbreaker/Reaxys/Reaxys_biocatalysis |
Top-N result to add to graph | 6 |
Feasible Synthetic Routes
Q1: What is the molecular formula of tetrathiafulvalene?
A1: The molecular formula of tetrathiafulvalene is C6H4S4.
Q2: What is the molecular weight of tetrathiafulvalene?
A2: The molecular weight of tetrathiafulvalene is 204.36 g/mol.
Q3: What are some key spectroscopic characteristics of tetrathiafulvalene derivatives?
A3: Tetrathiafulvalene derivatives are often characterized using techniques such as 1H NMR, 13C NMR, FT-IR, and mass spectrometry. [] Additionally, UV-Vis spectroelectrochemistry is useful for analyzing their redox behavior. []
Q4: How does the planarity of tetrathiafulvalene derivatives impact their properties?
A4: Planar tetrathiafulvalene derivatives tend to form stronger charge-transfer complexes and exhibit higher electrical conductivity. [] This is because planarity allows for better orbital overlap and more efficient charge transport.
Q5: What is a key characteristic of tetrathiafulvalene's electrochemical behavior?
A5: Tetrathiafulvalene is known for its strong electron-donating ability and undergoes two reversible, single-electron redox waves. []
Q6: Why is tetrathiafulvalene considered a good electron donor?
A6: The central C=C bond in tetrathiafulvalene is electron-rich due to the presence of four electron-donating sulfur atoms. This makes it easy for the molecule to lose electrons and form stable radical cation species.
Q7: What happens when tetrathiafulvalene interacts with an electron acceptor like tetracyanoquinodimethane (tetracyanoquinodimethane)?
A7: Tetrathiafulvalene forms charge-transfer complexes with electron acceptors like tetracyanoquinodimethane. [] These complexes often exhibit interesting electrical conductivity properties.
Q8: How does solvent polarity affect the charge-transfer properties of tetrathiafulvalene?
A8: The energy of the absorption maximum of the charge-transfer complex between tetrathiafulvalene derivatives and tetracyanoquinodimethane generally increases with decreasing solvent polarity. []
Q9: Can the oxidation potential of tetrathiafulvalene be tuned?
A9: Yes, the oxidation potential of tetrathiafulvalene can be tuned by introducing different substituents on the core structure. For instance, electron-withdrawing groups tend to increase the oxidation potential. [, ]
Q10: What are some applications of tetrathiafulvalene and its derivatives?
A10: Tetrathiafulvalene derivatives have found applications in various fields, including:
- Organic conductors and semiconductors: Their ability to form charge-transfer complexes and exhibit high electrical conductivity makes them suitable for use in organic field-effect transistors (OFETs), sensors, and other electronic devices. [, , ]
- Molecular electronics: Tetrathiafulvalene units are incorporated into larger molecular architectures to create molecular wires, switches, and other nanoscale electronic components. []
- Supramolecular chemistry: The redox activity of tetrathiafulvalene can be exploited for the development of molecular sensors and switches that respond to specific analytes or stimuli. []
- Chemiluminescence probes: Some tetrathiafulvalene derivatives have been developed as highly selective and sensitive chemiluminescence probes for singlet oxygen, finding use in chemical and biological systems. []
- Iodine capture: Tetrathiafulvalene-based covalent organic frameworks (COFs) with high surface areas and abundant tetrathiafulvalene groups demonstrate excellent iodine adsorption capacity, relevant for nuclear energy safety and environmental remediation. []
Q11: What factors influence the performance of tetrathiafulvalene-based materials in OFETs?
A11: Device performance is influenced by factors such as:
- Molecular packing: Closer stacking of molecules, often observed in specific polymorphs, can lead to higher charge carrier mobilities. []
- Substituent effects: Electron-withdrawing substituents can improve device stability and influence charge transport properties. [, ]
- Contact resistance: Minimizing contact resistance between the organic semiconductor and the electrodes is crucial for achieving high-performance devices. []
Q12: How does the choice of metal ion affect the properties of tetrathiafulvalene-metal complexes?
A12: The metal coordination center can significantly impact the properties of the complex. For example, in tetrathiafulvalene-metal complex dyads, redox-active metal centers like Ni(II), Cu(I), and Mn(II) can enhance the photocurrent response compared to the free ligand. []
Q13: How do tetrathiafulvalene-based COFs achieve high iodine capture capacity?
A13: These COFs combine both physical and chemical adsorption mechanisms. Their high specific surface areas facilitate physisorption, while the abundant tetrathiafulvalene groups provide strong chemisorption sites through interactions with iodine. []
Q14: How does modifying the tetrathiafulvalene core structure affect its electron-donating ability?
A14: Introducing electron-donating groups, such as alkyl or alkoxy substituents, generally enhances the electron-donating ability of tetrathiafulvalene. Conversely, electron-withdrawing groups, such as halogens or cyano groups, tend to reduce the electron-donating ability. [, ]
Q15: How does the length of alkyl chains in tetrathiafulvalene derivatives impact their self-assembly?
A15: Longer alkyl chains can promote self-assembly into ordered structures, such as lamellar phases, due to increased van der Waals interactions between the chains. This self-assembly can significantly influence the material's charge transport properties. []
Q16: How does the incorporation of a pyridyl group affect the sensing properties of tetrathiafulvalene?
A16: Ethylenedioxytetrathiafulvalene substituted with a pyridinyl group exhibits selective recognition and coordination towards Zn2+ ions, leading to significant changes in its UV-Vis absorption spectrum and redox properties. This highlights the potential of tetrathiafulvalene derivatives as selective chemosensors. []
Q17: What computational methods are commonly used to study tetrathiafulvalene and its derivatives?
A17: Density functional theory (DFT) calculations are frequently employed to investigate the electronic structure, redox potentials, and other properties of tetrathiafulvalene derivatives. [, , , , , ] Time-dependent DFT (TD-DFT) is used to study excited states and optical properties. []
Q18: What insights can DFT calculations provide about the charge transport properties of tetrathiafulvalene derivatives?
A18: DFT calculations can be used to estimate the reorganization energy, which is a key parameter for charge transport in organic semiconductors. Lower reorganization energies generally correspond to better charge transport properties. []
Q19: Can computational methods be used to predict the selectivity of tetrathiafulvalene-based sensors?
A19: Yes, DFT calculations, particularly those focusing on binding energies and molecular electrostatic potentials, can provide valuable insights into the selectivity of tetrathiafulvalene-based receptors towards specific analytes.
Q20: How stable are tetrathiafulvalene derivatives under ambient conditions?
A20: The stability of tetrathiafulvalene derivatives can vary depending on their substituents and the surrounding environment. Generally, they are more stable in their neutral state compared to their oxidized forms. []
Q21: How can the stability of tetrathiafulvalene-based materials be enhanced?
A21: Strategies for enhancing stability include:
- Incorporating electron-withdrawing groups: These groups can stabilize the highest occupied molecular orbital (HOMO) level, making the material less prone to oxidation. []
- Encapsulation in a protective matrix: Embedding the material in a polymer matrix can provide physical protection against environmental degradation. []
Q22: What are common synthetic routes for preparing tetrathiafulvalene derivatives?
A22: Several synthetic approaches have been developed, including:
- Wittig-type reactions: These reactions involve the condensation of a phosphonium ylide with a 1,3-dithiole-2-one or 1,3-dithiole-2-thione. [, , , ]
- Coupling reactions: Tetrathiafulvalene derivatives can be synthesized via the coupling of appropriately substituted 1,3-dithiole-2-thiones using reagents like triethyl phosphite or trimethyl phosphite. [, , ]
- Cross-coupling reactions: The reaction of tetrathiafulvalenylzinc derivatives with various electrophiles allows the introduction of diverse substituents onto the tetrathiafulvalene core. []
Q23: What is the role of protecting groups in the synthesis of functionalized tetrathiafulvalene derivatives?
A23: Protecting groups, such as the 2-cyanoethyl group, are commonly employed to mask reactive functional groups during synthesis. These groups can be selectively removed later to unveil the desired functionality without affecting the tetrathiafulvalene core. []
Descargo de responsabilidad e información sobre productos de investigación in vitro
Tenga en cuenta que todos los artículos e información de productos presentados en BenchChem están destinados únicamente con fines informativos. Los productos disponibles para la compra en BenchChem están diseñados específicamente para estudios in vitro, que se realizan fuera de organismos vivos. Los estudios in vitro, derivados del término latino "in vidrio", involucran experimentos realizados en entornos de laboratorio controlados utilizando células o tejidos. Es importante tener en cuenta que estos productos no se clasifican como medicamentos y no han recibido la aprobación de la FDA para la prevención, tratamiento o cura de ninguna condición médica, dolencia o enfermedad. Debemos enfatizar que cualquier forma de introducción corporal de estos productos en humanos o animales está estrictamente prohibida por ley. Es esencial adherirse a estas pautas para garantizar el cumplimiento de los estándares legales y éticos en la investigación y experimentación.