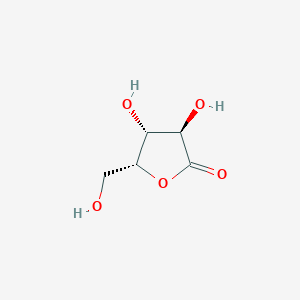
D-Xylono-1,4-lactone
Descripción general
Descripción
D-Xylono-1,4-lactone (CAS 18423-66-0) is a six-membered cyclic sugar lactone derived from D-xylose, a pentose sugar commonly sourced from renewable biomass like corn stover or sugarcane bagasse . It exists as a white crystalline solid with a melting point of 182–187°C, high water solubility, and stability under acidic and oxidative conditions . Its synthesis involves catalytic oxidation of D-xylose using copper(II) sulfate or cerium(IV) ammonium nitrate . Key applications include:
- Intermediate in synthesizing bioactive compounds (e.g., flavonoids, phenolic acids) .
- Precursor for xylitol, a low-calorie sweetener .
- Role in bacterial xylose catabolism, where it is hydrolyzed to xylonate by enzymes like xylose dehydrogenase (XD) and lactonases .
Analytical characterization employs HPLC, NMR, and GC, though distinguishing it from its open-chain acid (xylonic acid) requires specialized methods like the hydroxamate assay .
Métodos De Preparación
Synthetic Routes and Reaction Conditions: D-Xylono-1,4-lactone can be synthesized through the oxidation of D-xylose. The process involves the use of oxidizing agents such as bromine or chromium (VI) reagents. The reaction typically takes place in an aqueous medium, and the product is isolated by crystallization .
Industrial Production Methods: In industrial settings, this compound is produced through the fermentation of D-xylose using specific strains of bacteria or fungi. The fermentation broth is then subjected to purification processes, including filtration, concentration, and crystallization, to obtain the final product .
Análisis De Reacciones Químicas
Types of Reactions: D-Xylono-1,4-lactone undergoes various chemical reactions, including:
Oxidation: It can be oxidized to form D-xylonic acid.
Reduction: It can be reduced to form D-xylitol.
Hydrolysis: It can be hydrolyzed to form D-xylonic acid.
Common Reagents and Conditions:
Oxidation: Common oxidizing agents include bromine and chromium (VI) reagents.
Reduction: Sodium borohydride is commonly used as a reducing agent.
Hydrolysis: Acidic or basic conditions can facilitate the hydrolysis of this compound.
Major Products Formed:
Oxidation: D-xylonic acid
Reduction: D-xylitol
Hydrolysis: D-xylonic acid
Aplicaciones Científicas De Investigación
D-Xylono-1,4-lactone has several applications in scientific research:
Chemistry: It is used as a precursor for the synthesis of various organic compounds.
Biology: It serves as a substrate for studying enzyme activities, particularly lactonases.
Medicine: It is investigated for its potential role in metabolic pathways and its effects on cellular processes.
Industry: It is used in the production of biodegradable polymers and other environmentally friendly materials.
Mecanismo De Acción
D-Xylono-1,4-lactone exerts its effects primarily through its interaction with specific enzymes, such as lactonases. These enzymes catalyze the hydrolysis of this compound to D-xylonic acid. The reaction involves the activation of a water molecule, which then attacks the lactone ring, leading to its cleavage .
Comparación Con Compuestos Similares
Comparison with Structurally Similar Lactones
Structural and Functional Differences
Key Research Findings
- Enzymatic Reactivity: this compound is hydrolyzed 100× faster than D-glucono-1,5-lactone by the nonheme iron enzyme Cc XylC, highlighting the impact of ring size (1,4 vs. 1,5) and stereochemistry on catalytic efficiency .
- Biological Pathways: this compound is a key intermediate in xylose catabolism in Caulobacter crescentus, diverging from pathways like the XI/XDH route . L-Gulono-1,4-lactone and L-galactono-1,4-lactone are essential for vitamin C synthesis in mammals and plants, respectively, underscoring species-specific metabolic adaptations .
- Analytical Challenges: D-Threono-1,4-lactone and its open-chain acid (D-threonate) co-elute in HPLC, requiring alternative resolution methods (e.g., hydroxamate assay) .
Actividad Biológica
D-Xylono-1,4-lactone is a biochemical compound that has garnered attention for its potential biological activities, particularly in enzymatic hydrolysis processes. This article explores the biological activity of this compound, focusing on its enzymatic interactions, structural characteristics, and implications in metabolic pathways.
- Molecular Formula : CHO
- Molecular Weight : 148.114 g/mol
- CAS Number : 18423-66-0
This compound is recognized as a biochemical reagent in various life science research applications .
Enzymatic Hydrolysis
This compound serves as a substrate for specific enzymes, particularly lactonases. These enzymes catalyze the hydrolysis of the lactone ring, leading to the formation of D-xylonate. The following sections detail key studies that elucidate the enzymatic activity associated with this compound.
Key Enzymes Involved
- Xylonolactonase from Caulobacter crescentus :
-
Lactonases from Mycoplasma species :
- Enzymes such as MS53_0025 and MAG_6390 have been characterized to hydrolyze this compound-5-phosphate with high catalytic efficiency (k/K values around ) .
- These enzymes are implicated in the catabolism of small sugar lactones, suggesting a metabolic pathway that utilizes this compound as an energy source.
Structural Characterization
The structural analysis of this compound and its interacting enzymes provides insights into their functional mechanisms:
Enzyme | Structure Type | Active Site Composition | Metal Ion |
---|---|---|---|
Xylonolactonase (Cc XylC) | 6-bladed β-propeller | Glu18, Asn146, Asp196 | Fe |
Lactonases (MS53_0025) | Amidohydrolase fold | Binuclear zinc center | Zn |
The crystal structures reveal that the active sites of these enzymes are well-suited for binding to this compound, facilitating efficient hydrolysis .
Metabolic Pathways
This compound is involved in several metabolic pathways. Notably, it can be converted into D-xylonate through enzymatic action. The oxidative D-xylose pathway has been identified as a significant route for producing organic acids and alcohols from biomass-derived sugars . This pathway highlights the potential for biotechnological applications in converting renewable resources into valuable chemicals.
Case Studies
-
Metabolic Engineering of Saccharomyces cerevisiae :
- A study demonstrated that expressing an NAD-dependent D-xylose dehydrogenase from Caulobacter crescentus in yeast led to significant production of D-xylonate from D-xylose. This indicates the feasibility of utilizing this compound derivatives in engineered microbial systems for organic acid production .
- Hydrolysis Kinetics :
Q & A
Basic Research Questions
Q. What are the established methods for synthesizing D-Xylono-1,4-lactone, and how do they differ in yield and purity?
this compound is synthesized via enzymatic oxidation of xylose using xylose dehydrogenase (XD) with NAD⁺/NADP⁺ cofactors, followed by lactonization. Acid-catalyzed lactone formation from D-xylonate is also employed, achieving ~65% conversion under 0.1 M HCl at 95°C for 3 hours . Yield optimization requires pH control, as spontaneous hydrolysis competes with lactone formation. Purity assessment typically involves HPLC or NMR to distinguish between 1,4- and 1,5-lactone isomers .
Q. How is the toxicity profile of this compound evaluated in vitro, and what are the recommended safety protocols?
Toxicity is assessed using cell viability assays (e.g., MTT) on normal cell lines, confirming low cytotoxicity at concentrations below 10 mM. Safety protocols include handling in ventilated hoods, using personal protective equipment (PPE), and avoiding prolonged inhalation. While no acute toxicity is reported, MSDS guidelines recommend emergency washing and medical consultation upon exposure .
Q. What analytical techniques are standard for characterizing this compound structure and stability?
Key methods include:
- ¹H/¹³C NMR : To confirm lactone ring conformation (e.g., envelope vs. chair) and monitor hydrolysis kinetics .
- Mass spectrometry : For molecular weight validation (148.11 g/mol) and detecting degradation products .
- Polarimetry : To measure optical rotation ([α]/D values) and assess enantiomeric purity . Stability studies use pH-controlled buffers and thermal gravimetric analysis (TGA) to evaluate decomposition thresholds .
Advanced Research Questions
Q. How do enzymatic and non-enzymatic pathways for this compound hydrolysis compare in catalytic efficiency?
The enzyme xylonolactonase (e.g., from Caulobacter crescentus) accelerates hydrolysis 100-fold via a mononuclear Fe²⁺-dependent mechanism, achieving kcat/Km values of 4.7 × 10⁴ M⁻¹s⁻¹ for this compound-5-phosphate . Non-enzymatic hydrolysis relies on acid/base catalysis, with rate constants pH-dependent and slower by 1–2 orders of magnitude . Competitive inhibition studies using L-arabino-1,4-lactone-5-phosphate reveal substrate specificity nuances .
Q. What experimental strategies resolve contradictions in reported biological activities of this compound?
Discrepancies in bioactivity (e.g., anti-inflammatory vs. inert effects) arise from lactone stability and isomerization. Strategies include:
- Strict pH control : To minimize spontaneous hydrolysis to xylonate during assays .
- Isomer-specific activity assays : Separating 1,4- and 1,5-lactones via chiral chromatography .
- Gene knockout models : To isolate lactone-specific effects in metabolic pathways (e.g., ascorbate biosynthesis) .
Q. How can this compound be tracked in vivo, and what metabolic pathways are implicated?
Isotopic labeling (¹³C or ²H) combined with LC-MS/MS tracks lactone uptake and conversion. In Arabidopsis, this compound intersects with L-gulose and myo-inositol pathways, contributing to ascorbate synthesis via L-gulono-1,4-lactone oxidase (GulLO) . Metabolomic heatmaps (KEGG enrichment) identify downstream products like gluconic acid and succinate in microbial systems .
Q. What methodologies optimize this compound’s solubility for industrial biocatalysis?
Solubility challenges in organic solvents are addressed via:
- Co-solvent systems : Using DMSO/water mixtures to enhance solubility without denaturing enzymes .
- Ionic liquid matrices : Imidazolium-based liquids improve stability during xylitol synthesis .
- Nanoencapsulation : Liposomal carriers increase bioavailability in pharmaceutical applications .
Q. How does the binuclear metal center in lactonases influence substrate selectivity for this compound derivatives?
Crystal structures (e.g., MS53_0025 lactonase at 2.06 Å resolution) show a Zn²⁺/Fe²⁺ cluster that coordinates phosphate groups in this compound-5-phosphate. Mutagenesis of residues (e.g., Lys217, Arg275) disrupts H-bonding to the lactone carbonyl, reducing kcat/Km by >90% . Molecular docking simulations prioritize substrates with C5-phosphate moieties over unmodified lactones .
Q. Methodological Notes
- References : Prioritize primary literature (e.g., Carbohydrate Research, Journal of Biological Chemistry) over commercial databases.
- Data Reproducibility : Detailed experimental protocols (buffer conditions, enzyme sources) are critical for replication .
- Open Access Tools : Use Phaser-2.1 for crystallographic analysis and KEGG for pathway mapping .
Propiedades
IUPAC Name |
(3R,4R,5R)-3,4-dihydroxy-5-(hydroxymethyl)oxolan-2-one | |
---|---|---|
Source | PubChem | |
URL | https://pubchem.ncbi.nlm.nih.gov | |
Description | Data deposited in or computed by PubChem | |
InChI |
InChI=1S/C5H8O5/c6-1-2-3(7)4(8)5(9)10-2/h2-4,6-8H,1H2/t2-,3+,4-/m1/s1 | |
Source | PubChem | |
URL | https://pubchem.ncbi.nlm.nih.gov | |
Description | Data deposited in or computed by PubChem | |
InChI Key |
CUOKHACJLGPRHD-FLRLBIABSA-N | |
Source | PubChem | |
URL | https://pubchem.ncbi.nlm.nih.gov | |
Description | Data deposited in or computed by PubChem | |
Canonical SMILES |
C(C1C(C(C(=O)O1)O)O)O | |
Source | PubChem | |
URL | https://pubchem.ncbi.nlm.nih.gov | |
Description | Data deposited in or computed by PubChem | |
Isomeric SMILES |
C([C@@H]1[C@@H]([C@H](C(=O)O1)O)O)O | |
Source | PubChem | |
URL | https://pubchem.ncbi.nlm.nih.gov | |
Description | Data deposited in or computed by PubChem | |
Molecular Formula |
C5H8O5 | |
Source | PubChem | |
URL | https://pubchem.ncbi.nlm.nih.gov | |
Description | Data deposited in or computed by PubChem | |
DSSTOX Substance ID |
DTXSID801315147 | |
Record name | D-Xylono-1,4-lactone | |
Source | EPA DSSTox | |
URL | https://comptox.epa.gov/dashboard/DTXSID801315147 | |
Description | DSSTox provides a high quality public chemistry resource for supporting improved predictive toxicology. | |
Molecular Weight |
148.11 g/mol | |
Source | PubChem | |
URL | https://pubchem.ncbi.nlm.nih.gov | |
Description | Data deposited in or computed by PubChem | |
CAS No. |
15384-37-9 | |
Record name | D-Xylono-1,4-lactone | |
Source | CAS Common Chemistry | |
URL | https://commonchemistry.cas.org/detail?cas_rn=15384-37-9 | |
Description | CAS Common Chemistry is an open community resource for accessing chemical information. Nearly 500,000 chemical substances from CAS REGISTRY cover areas of community interest, including common and frequently regulated chemicals, and those relevant to high school and undergraduate chemistry classes. This chemical information, curated by our expert scientists, is provided in alignment with our mission as a division of the American Chemical Society. | |
Explanation | The data from CAS Common Chemistry is provided under a CC-BY-NC 4.0 license, unless otherwise stated. | |
Record name | D-Xylono-1,4-lactone | |
Source | EPA DSSTox | |
URL | https://comptox.epa.gov/dashboard/DTXSID801315147 | |
Description | DSSTox provides a high quality public chemistry resource for supporting improved predictive toxicology. | |
Retrosynthesis Analysis
AI-Powered Synthesis Planning: Our tool employs the Template_relevance Pistachio, Template_relevance Bkms_metabolic, Template_relevance Pistachio_ringbreaker, Template_relevance Reaxys, Template_relevance Reaxys_biocatalysis model, leveraging a vast database of chemical reactions to predict feasible synthetic routes.
One-Step Synthesis Focus: Specifically designed for one-step synthesis, it provides concise and direct routes for your target compounds, streamlining the synthesis process.
Accurate Predictions: Utilizing the extensive PISTACHIO, BKMS_METABOLIC, PISTACHIO_RINGBREAKER, REAXYS, REAXYS_BIOCATALYSIS database, our tool offers high-accuracy predictions, reflecting the latest in chemical research and data.
Strategy Settings
Precursor scoring | Relevance Heuristic |
---|---|
Min. plausibility | 0.01 |
Model | Template_relevance |
Template Set | Pistachio/Bkms_metabolic/Pistachio_ringbreaker/Reaxys/Reaxys_biocatalysis |
Top-N result to add to graph | 6 |
Feasible Synthetic Routes
Descargo de responsabilidad e información sobre productos de investigación in vitro
Tenga en cuenta que todos los artículos e información de productos presentados en BenchChem están destinados únicamente con fines informativos. Los productos disponibles para la compra en BenchChem están diseñados específicamente para estudios in vitro, que se realizan fuera de organismos vivos. Los estudios in vitro, derivados del término latino "in vidrio", involucran experimentos realizados en entornos de laboratorio controlados utilizando células o tejidos. Es importante tener en cuenta que estos productos no se clasifican como medicamentos y no han recibido la aprobación de la FDA para la prevención, tratamiento o cura de ninguna condición médica, dolencia o enfermedad. Debemos enfatizar que cualquier forma de introducción corporal de estos productos en humanos o animales está estrictamente prohibida por ley. Es esencial adherirse a estas pautas para garantizar el cumplimiento de los estándares legales y éticos en la investigación y experimentación.