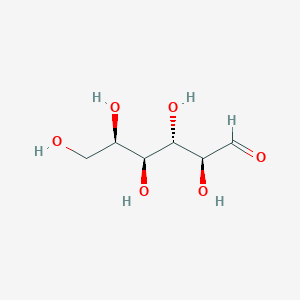
D-Talose
- Haga clic en CONSULTA RÁPIDA para recibir una cotización de nuestro equipo de expertos.
- Con productos de calidad a un precio COMPETITIVO, puede centrarse más en su investigación.
Descripción general
Descripción
Aldehydo-D-talose is the acyclic form of D-Talose . It is a natural product found in Pogostemon cablin . This compound, a C-2 epimer of galactose, is used as a substrate to identify, differentiate and characterize ribose-5-phosphate isomerase(s) of Clostridia .
Molecular Structure Analysis
The molecular formula of aldehydo-D-talose is C6H12O6 . The molecular weight is 180.16 g/mol .Physical And Chemical Properties Analysis
The molecular weight of aldehydo-D-talose is 180.16 g/mol . The exact mass is 180.06338810 g/mol . It has a hydrogen bond donor count of 5 and a hydrogen bond acceptor count of 6 . It has a rotatable bond count of 5 .Aplicaciones Científicas De Investigación
NMR Spectroscopy and Tautomeric Equilibria
Aldehydo-D-talose has been studied using high-resolution NMR spectroscopy to quantify its acyclic forms in aqueous solutions. This research aids in understanding the tautomeric equilibria and the influence of deuterium isotope effects on the interconversion of cyclic forms of aldohexoses like D-talose (Zhu, Zajicek, & Serianni, 2001).
Oxidation and Degradation Studies
This compound has been studied for its reaction with sodium peroxide, providing insights into the stepwise degradation of aldoses to formic acid. This research is vital for understanding the chemical processes involving carbohydrates (Isbell, Frush, & Martin, 1973).
Synthesis of Derivatives
Research on the synthesis of 3-deoxyoctitols from this compound provides important data for the development of novel compounds. These findings are significant in the identification of constituents in natural products such as lipopolysaccharides of various bacteria (Krulle, Schmidt, Brade, & Holst, 1993).
Biochemical and Biological Studies
This compound has been observed to exhibit growth inhibitory effects against the nematode Caenorhabditis elegans. This research highlights the potential biological and biochemical applications of rare sugars like this compound in studying model organisms (Sakoguchi, Yoshihara, Izumori, & Sato, 2016).
Enzymatic Synthesis Pathways
Studies on the enzymatic pathways for synthesizing GDP-6-deoxy-D-talose in bacteria like Actinobacillus actinomycetemcomitans have been conducted. This research is crucial for understanding the biosynthesis of microbial polysaccharides and their unique sugar constituents (Suzuki et al., 2002).
Synthesis of Protected Rare Sugars
Efficient methodologies have been developed for synthesizing orthogonally protected rare sugars like L-talose, which is significant for creating complex carbohydrates in pharmaceutical and biotechnological applications (Dibello, Gamenara, & Seoane, 2016).
Conversion of Galactose to Talose
Research on the conversion of D-galactose to this compound using enzymes like cellobiose 2-epimerase reveals significant biotechnological applications in producing rare sugars with antimicrobial and anti-inflammatory properties (Van Overtveldt et al., 2018).
Mecanismo De Acción
Safety and Hazards
Direcciones Futuras
There is growing interest in low-temperature ALD and ALD-inspired processes for newer and more wide-ranging applications, including integration with biological and synthetic polymer structures . The physiological effects of many rare sugars have been extensively studied, including D-form and L-form rare sugars and some sugar alcohols .
Propiedades
{ "Design of the Synthesis Pathway": "The synthesis of aldehydo-D-talose can be achieved through a multistep process starting from D-glucose. The first step involves the oxidation of D-glucose to D-glucuronic acid, which is then converted to D-glucono-1,5-lactone. The lactone is then hydrolyzed to form D-gluconic acid, which is further oxidized to D-gluconaldehyde. Finally, D-gluconaldehyde is reduced to aldehydo-D-talose.", "Starting Materials": [ "D-glucose", "Oxidizing agent (e.g. nitric acid, sodium chlorite)", "Hydrolyzing agent (e.g. sodium hydroxide)", "Reducing agent (e.g. sodium borohydride)" ], "Reaction": [ "D-glucose is oxidized to D-glucuronic acid using an oxidizing agent.", "D-glucuronic acid is converted to D-glucono-1,5-lactone through intramolecular esterification.", "D-glucono-1,5-lactone is hydrolyzed to D-gluconic acid using a hydrolyzing agent.", "D-gluconic acid is oxidized to D-gluconaldehyde using an oxidizing agent.", "D-gluconaldehyde is reduced to aldehydo-D-talose using a reducing agent." ] } | |
Número CAS |
2595-98-4 |
Fórmula molecular |
C6H12O6 |
Peso molecular |
180.16 g/mol |
Nombre IUPAC |
(3S,4S,5R,6R)-6-(hydroxymethyl)oxane-2,3,4,5-tetrol |
InChI |
InChI=1S/C6H12O6/c7-1-2-3(8)4(9)5(10)6(11)12-2/h2-11H,1H2/t2-,3+,4+,5+,6?/m1/s1 |
Clave InChI |
WQZGKKKJIJFFOK-WHZQZERISA-N |
SMILES isomérico |
C([C@@H]1[C@@H]([C@@H]([C@@H](C(O1)O)O)O)O)O |
SMILES |
C(C(C(C(C(C=O)O)O)O)O)O |
SMILES canónico |
C(C1C(C(C(C(O1)O)O)O)O)O |
Sinónimos |
D(+)-Talose; NSC 224293; |
Origen del producto |
United States |
Retrosynthesis Analysis
AI-Powered Synthesis Planning: Our tool employs the Template_relevance Pistachio, Template_relevance Bkms_metabolic, Template_relevance Pistachio_ringbreaker, Template_relevance Reaxys, Template_relevance Reaxys_biocatalysis model, leveraging a vast database of chemical reactions to predict feasible synthetic routes.
One-Step Synthesis Focus: Specifically designed for one-step synthesis, it provides concise and direct routes for your target compounds, streamlining the synthesis process.
Accurate Predictions: Utilizing the extensive PISTACHIO, BKMS_METABOLIC, PISTACHIO_RINGBREAKER, REAXYS, REAXYS_BIOCATALYSIS database, our tool offers high-accuracy predictions, reflecting the latest in chemical research and data.
Strategy Settings
Precursor scoring | Relevance Heuristic |
---|---|
Min. plausibility | 0.01 |
Model | Template_relevance |
Template Set | Pistachio/Bkms_metabolic/Pistachio_ringbreaker/Reaxys/Reaxys_biocatalysis |
Top-N result to add to graph | 6 |
Feasible Synthetic Routes
Descargo de responsabilidad e información sobre productos de investigación in vitro
Tenga en cuenta que todos los artículos e información de productos presentados en BenchChem están destinados únicamente con fines informativos. Los productos disponibles para la compra en BenchChem están diseñados específicamente para estudios in vitro, que se realizan fuera de organismos vivos. Los estudios in vitro, derivados del término latino "in vidrio", involucran experimentos realizados en entornos de laboratorio controlados utilizando células o tejidos. Es importante tener en cuenta que estos productos no se clasifican como medicamentos y no han recibido la aprobación de la FDA para la prevención, tratamiento o cura de ninguna condición médica, dolencia o enfermedad. Debemos enfatizar que cualquier forma de introducción corporal de estos productos en humanos o animales está estrictamente prohibida por ley. Es esencial adherirse a estas pautas para garantizar el cumplimiento de los estándares legales y éticos en la investigación y experimentación.