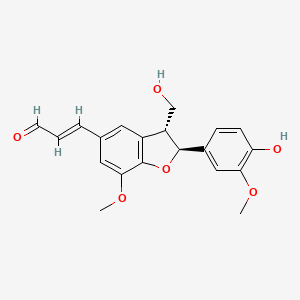
Balanophonin
- Haga clic en CONSULTA RÁPIDA para recibir una cotización de nuestro equipo de expertos.
- Con productos de calidad a un precio COMPETITIVO, puede centrarse más en su investigación.
Descripción general
Descripción
Balanophonin is a neo-lignan . It is a bioactive compound that can be isolated from Dipteryx odorata and Balanophora japonica . It has been reported to have anti-inflammatory and anti-cancer effects .
Synthesis Analysis
The structure and stereochemistry of Balanophonin were determined by a combination of chemical methods and extensive use of 1H and 13C-NMR spectrometry .Molecular Structure Analysis
Balanophonin has a molecular formula of C20H20O6 . It is a phenolic compound .Chemical Reactions Analysis
Balanophonin possesses anti-oxidant, anticholinesterase, anti-inflammatory, anticancer, and antineurodegenerative activities .Physical And Chemical Properties Analysis
Balanophonin has a molar mass of 356.37 g/mol . The standard state data are given for materials at 25 °C [77 °F], 100 kPa .Aplicaciones Científicas De Investigación
Anti-Inflammatory Activities
Balanophonin has been found to have anti-inflammatory properties. In a study conducted on the seeds of Lithocarpus pachylepis, twelve phenolics, including balanophonin, were isolated . These compounds were evaluated for their anti-inflammatory activities on lipopolysaccharide (LPS)-induced nitric oxide (NO) production in RAW 264.7 and showed moderate inhibitory activities, with IC 50 values ranging from 10.9 to 34.7 μM .
Neuroprotective Effects
Balanophonin, a natural neolignoid from Firmiana simplex, has been reported to have anti-neuroinflammatory effects . In a study, the anti-neuroinflammatory effects and mechanism of balanophonin in lipopolysaccharide (LPS)-stimulated BV2 microglia cells were evaluated . The results indicated that balanophonin reduced not only the LPS-mediated TLR4 activation but also the production of inflammatory mediators, such as nitric oxide (NO), prostaglandin E2 (PGE2), Interleukin-1β (IL-1β), and tumor necrosis factor-α (TNF-α), in BV2 cells . Balanophonin also inhibited LPS-induced inducible nitric oxide synthase (iNOS), and cyclooxygenase-2 (COX2) protein expression and mitogen activated protein kinases (MAPKs), including extracellular signal-regulated kinase (ERK1/2), c-Jun N-terminal kinase (JNK), and p38 MAPK . Interestingly, it also inhibited neuronal cell death resulting from LPS-activated microglia by regulating cleaved caspase-3 and poly ADP ribose polymerase (PARP) cleavage in N2a cells .
Mecanismo De Acción
Target of Action
Balanophonin primarily targets microglia cells . Microglia are key immune cells in the brain that respond to emergency situations such as brain injury or immunological conditions .
Mode of Action
Balanophonin interacts with its targets by inhibiting their activation .
Biochemical Pathways
Balanophonin affects several biochemical pathways. It inhibits the production of inflammatory mediators such as nitric oxide (NO), prostaglandin E2 (PGE2), Interleukin-1β (IL-1β), and tumor necrosis factor-α (TNF-α) in BV2 cells . It also inhibits LPS-induced inducible nitric oxide synthase (iNOS), and cyclooxygenase-2 (COX2) protein expression . Furthermore, it affects the mitogen-activated protein kinases (MAPKs), including extracellular signal-regulated kinase (ERK1/2), c-Jun N-terminal kinase (JNK), and p38 MAPK .
Result of Action
The molecular and cellular effects of Balanophonin’s action are significant. It inhibits neuronal cell death resulting from LPS-activated microglia by regulating cleaved caspase-3 and poly ADP ribose polymerase (PARP) cleavage in N2a cells . This suggests that Balanophonin may delay the progression of neuronal cell death by inhibiting microglial activation .
Action Environment
It’s worth noting that balanophonin is a natural compound isolated from plants that grow in tropical and subtropical regions of asia and oceania . The specific environmental conditions in these regions may play a role in the compound’s properties and effects.
Safety and Hazards
Balanophonin should be handled with care. Avoid dust formation and avoid breathing mist, gas, or vapors. Avoid contacting with skin and eye. Use personal protective equipment. Wear chemical impermeable gloves. Ensure adequate ventilation. Remove all sources of ignition. Evacuate personnel to safe areas. Keep people away from and upwind of spill/leak .
Propiedades
IUPAC Name |
(E)-3-[(2S,3R)-2-(4-hydroxy-3-methoxyphenyl)-3-(hydroxymethyl)-7-methoxy-2,3-dihydro-1-benzofuran-5-yl]prop-2-enal |
Source
|
---|---|---|
Source | PubChem | |
URL | https://pubchem.ncbi.nlm.nih.gov | |
Description | Data deposited in or computed by PubChem | |
InChI |
InChI=1S/C20H20O6/c1-24-17-10-13(5-6-16(17)23)19-15(11-22)14-8-12(4-3-7-21)9-18(25-2)20(14)26-19/h3-10,15,19,22-23H,11H2,1-2H3/b4-3+/t15-,19+/m0/s1 |
Source
|
Source | PubChem | |
URL | https://pubchem.ncbi.nlm.nih.gov | |
Description | Data deposited in or computed by PubChem | |
InChI Key |
GWCSSLSMGCFIFR-LNFBDUAVSA-N |
Source
|
Source | PubChem | |
URL | https://pubchem.ncbi.nlm.nih.gov | |
Description | Data deposited in or computed by PubChem | |
Canonical SMILES |
COC1=CC(=CC2=C1OC(C2CO)C3=CC(=C(C=C3)O)OC)C=CC=O |
Source
|
Source | PubChem | |
URL | https://pubchem.ncbi.nlm.nih.gov | |
Description | Data deposited in or computed by PubChem | |
Isomeric SMILES |
COC1=CC(=CC2=C1O[C@@H]([C@H]2CO)C3=CC(=C(C=C3)O)OC)/C=C/C=O |
Source
|
Source | PubChem | |
URL | https://pubchem.ncbi.nlm.nih.gov | |
Description | Data deposited in or computed by PubChem | |
Molecular Formula |
C20H20O6 |
Source
|
Source | PubChem | |
URL | https://pubchem.ncbi.nlm.nih.gov | |
Description | Data deposited in or computed by PubChem | |
Molecular Weight |
356.4 g/mol |
Source
|
Source | PubChem | |
URL | https://pubchem.ncbi.nlm.nih.gov | |
Description | Data deposited in or computed by PubChem | |
Product Name |
(+)-Balanophonin |
Q & A
Q1: How does balanophonin exert its anti-inflammatory effects?
A1: Balanophonin has been shown to inhibit the activation of TLR4, a receptor involved in the inflammatory response, in LPS-stimulated BV2 microglia cells []. This inhibition, in turn, reduces the production of inflammatory mediators such as nitric oxide (NO), prostaglandin E2 (PGE2), interleukin-1β (IL-1β), and tumor necrosis factor-α (TNF-α) [].
Q2: What is the significance of inhibiting microglial activation?
A2: Excessive activation of microglia, the resident immune cells of the central nervous system, contributes to neuroinflammation, a key player in the progression of neurodegenerative diseases. By inhibiting microglial activation, balanophonin may help protect neurons from inflammatory damage [].
Q3: Does balanophonin directly impact neuronal cell death?
A3: Research suggests that balanophonin can indirectly protect neuronal cells from death induced by LPS-activated microglia. This protection is linked to the regulation of cleaved caspase-3 and poly ADP ribose polymerase (PARP) cleavage in N2a cells [].
Q4: Has balanophonin demonstrated anti-inflammatory activity in other models?
A4: Yes, balanophonin, along with other phenolic compounds isolated from Lithocarpus pachylepis seeds, exhibited moderate inhibitory activities against lipopolysaccharide (LPS)-induced nitric oxide (NO) production in RAW 264.7 cells, a model for inflammation [].
Q5: What is the molecular formula and weight of balanophonin?
A5: The molecular formula of balanophonin is C20H22O6, and its molecular weight is 358.39 g/mol [].
Q6: What spectroscopic data is available for characterizing balanophonin?
A6: The structure of balanophonin has been elucidated using a variety of spectroscopic techniques, including UV, IR, MS, 1D and 2D NMR [, , ]. This data provides detailed information about the compound's structure and functional groups.
Q7: What are the natural sources of balanophonin?
A7: Balanophonin has been isolated from various plant species, including Firmiana simplex [], Cirsium species [], Cornus officinalis [], Neonauclea reticulata [], Passiflora edulis [], Lithocarpus pachylepis [], and Balanophora japonica [], among others.
Q8: Are there any studies on the stability of balanophonin under various conditions?
A8: Specific studies focusing on the stability of balanophonin under different conditions are currently limited in the available literature.
Q9: Have any formulation strategies been explored to enhance the stability, solubility, or bioavailability of balanophonin?
A9: Currently, there is limited information available regarding specific formulation strategies employed to enhance the stability, solubility, or bioavailability of balanophonin.
Q10: Have computational methods been used to study balanophonin?
A10: Yes, molecular docking studies have been performed using balanophonin. One study utilized Molegro Virtual Docker to assess its potential inhibitory activity against p38α mitogen-activated protein kinase (p38α MAPK) []. Another study investigated its potential as an α-amylase inhibitor through molecular docking with porcine pancreatic α-amylase [].
Descargo de responsabilidad e información sobre productos de investigación in vitro
Tenga en cuenta que todos los artículos e información de productos presentados en BenchChem están destinados únicamente con fines informativos. Los productos disponibles para la compra en BenchChem están diseñados específicamente para estudios in vitro, que se realizan fuera de organismos vivos. Los estudios in vitro, derivados del término latino "in vidrio", involucran experimentos realizados en entornos de laboratorio controlados utilizando células o tejidos. Es importante tener en cuenta que estos productos no se clasifican como medicamentos y no han recibido la aprobación de la FDA para la prevención, tratamiento o cura de ninguna condición médica, dolencia o enfermedad. Debemos enfatizar que cualquier forma de introducción corporal de estos productos en humanos o animales está estrictamente prohibida por ley. Es esencial adherirse a estas pautas para garantizar el cumplimiento de los estándares legales y éticos en la investigación y experimentación.