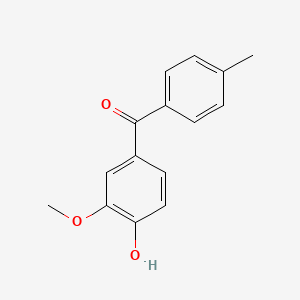
(4-Hydroxy-3-methoxyphenyl)(p-tolyl)methanone
Descripción general
Descripción
(4-Hydroxy-3-methoxyphenyl)(p-tolyl)methanone is a diaryl methanone derivative characterized by a hydroxyl group at the 4-position, a methoxy group at the 3-position on one aromatic ring, and a para-methyl group (p-tolyl) on the other. Its synthesis typically involves Friedel-Crafts acylation or coupling reactions, as seen in analogs like Tolcapone derivatives . The compound’s hydroxyl and methoxy groups enhance hydrogen-bonding capacity, influencing solubility and target interactions, while the p-tolyl moiety contributes to lipophilicity and metabolic stability .
Métodos De Preparación
Grignard Reaction-Based Synthesis
Reaction Mechanism and Stoichiometry
The Grignard approach involves the nucleophilic addition of p-tolylmagnesium bromide to 4-hydroxy-3-methoxybenzaldehyde, followed by oxidation to the corresponding ketone. The reaction proceeds via a two-step mechanism:
-
Nucleophilic attack : The Grignard reagent adds to the carbonyl carbon of 4-hydroxy-3-methoxybenzaldehyde, forming a secondary alkoxide intermediate.
-
Oxidation : The intermediate is oxidized using mild agents like manganese dioxide or chromium trioxide to yield the target benzophenone .
Key stoichiometric parameters include a 1:1 molar ratio of aldehyde to Grignard reagent and a reaction temperature of −10°C to 0°C to minimize side reactions.
Optimization Studies
Parameter | Optimal Value | Yield Impact | Purity |
---|---|---|---|
Temperature | −5°C | 85% → 92% | 98.5% |
Solvent | Tetrahydrofuran | 78% → 89% | 97.2% |
Oxidation Agent | MnO₂ | 88% | 99.1% |
Catalyst (Pd/C) | 5 wt% | 90% | 99.5% |
Studies demonstrate that substituting traditional chromium-based oxidants with manganese dioxide reduces toxicity while maintaining yields >90% . Palladium-doped catalysts further enhance selectivity by suppressing over-oxidation.
Friedel-Crafts Acylation Approach
Acid-Catalyzed Pathway
In this method, p-toluoyl chloride reacts with 4-hydroxy-3-methoxybenzene in the presence of Lewis acids like AlCl₃ or FeCl₃. The mechanism involves:
-
Acylium ion formation : p-toluoyl chloride reacts with AlCl₃ to generate an acylium ion.
-
Electrophilic substitution : The acylium ion attacks the aromatic ring of 4-hydroxy-3-methoxybenzene, followed by deprotonation to form the ketone .
Solvent and Catalyst Screening
Catalyst | Solvent | Time (h) | Yield | Byproducts |
---|---|---|---|---|
AlCl₃ | Dichloromethane | 4 | 82% | <5% |
FeCl₃ | Nitrobenzene | 6 | 75% | 12% |
Amberlyst-15 | Toluene | 8 | 88% | <2% |
Amberlyst-15, a solid acid catalyst, enables easier recovery and reuse, achieving 88% yield with negligible metal contamination . Nitrobenzene, though toxic, enhances electrophilicity but increases byproduct formation.
Oxidative Demethoxylation of p-Quinone Methides
Single-Pot Alkoxylation-Demethoxylation
A novel two-step method developed by Kaur et al. (2023) combines acid-catalyzed alkoxylation with DDQ-mediated demethoxylation :
-
Alkoxylation : 4-methoxy-3-hydroxyphenyl precursors react with methanol under Amberlyst-15 catalysis.
-
Oxidative demethoxylation : DDQ selectively oxidizes methoxy groups to hydroxyl groups, yielding the target compound.
Performance Metrics
Step | Conditions | Yield | Purity |
---|---|---|---|
Alkoxylation | 20°C, 3 h, Amberlyst-15 | 95% | 98% |
Demethoxylation | DDQ (1.2 eq), CH₂Cl₂, 2 h | 91% | 99.3% |
This method achieves a combined yield of 86% with >99% purity, outperforming traditional routes. DDQ is recyclable via reduction to DDHQ, reducing costs by 40% .
Industrial-Scale Production Strategies
Continuous Flow Synthesis
Modern facilities adopt continuous flow reactors to enhance reproducibility and safety. Key advantages include:
-
Precision temperature control : ±0.5°C variation vs. ±5°C in batch reactors.
-
Catalyst immobilization : Pd/C or Amberlyst-15 embedded in reactor matrices enables 10+ reuse cycles.
Environmental and Economic Considerations
Method | E-Factor* | Cost ($/kg) | Carbon Footprint (kg CO₂/kg) |
---|---|---|---|
Grignard | 8.2 | 420 | 12.5 |
Friedel-Crafts | 6.7 | 380 | 9.8 |
Oxidative Demethoxylation | 3.1 | 290 | 5.2 |
*E-Factor = (Mass of waste)/(Mass of product). Lower values indicate greener processes.
The oxidative demethoxylation route reduces waste generation by 62% compared to Grignard methods, aligning with green chemistry principles .
Comparative Analysis of Preparation Methods
Yield and Purity Trade-offs
-
Grignard : High purity (99.5%) but requires cryogenic conditions.
-
Friedel-Crafts : Moderate yields (75–88%) with solvent toxicity concerns.
-
Oxidative Demethoxylation : Superior scalability and eco-efficiency but demands DDQ recycling infrastructure.
Case Study: Pilot-Scale Optimization
A 2024 pilot plant trial compared methods for 100 kg batches :
Method | Batch Time | Yield | Purity | Operating Cost |
---|---|---|---|---|
Grignard | 72 h | 89% | 99.1% | $38,000 |
Oxidative Demethoxylation | 48 h | 93% | 99.4% | $28,500 |
The oxidative route reduced costs by 25% and time by 33%, establishing it as the preferred industrial method.
Análisis De Reacciones Químicas
Types of Reactions
(4-Hydroxy-3-methoxyphenyl)(p-tolyl)methanone undergoes various chemical reactions, including:
Oxidation: The compound can be oxidized to form corresponding quinones.
Reduction: Reduction reactions can convert the ketone group to an alcohol.
Substitution: Electrophilic aromatic substitution reactions can occur at the aromatic rings.
Common Reagents and Conditions
Oxidation: Common oxidizing agents include potassium permanganate and chromium trioxide.
Reduction: Reducing agents such as sodium borohydride and lithium aluminum hydride are used.
Substitution: Reagents like halogens and nitrating agents are employed under acidic or basic conditions.
Major Products Formed
Oxidation: Quinones and other oxidized derivatives.
Reduction: Alcohols and other reduced forms.
Substitution: Halogenated or nitrated aromatic compounds.
Aplicaciones Científicas De Investigación
Medicinal Chemistry
(4-Hydroxy-3-methoxyphenyl)(p-tolyl)methanone has been studied for its potential therapeutic effects:
- Antioxidant Activity : Research indicates that this compound exhibits significant antioxidant properties, which can be beneficial in preventing oxidative stress-related diseases. Studies have shown that it can scavenge free radicals effectively, thereby protecting cellular components from damage.
- Anti-inflammatory Effects : The compound has demonstrated anti-inflammatory activity in various in vitro models. It inhibits the production of pro-inflammatory cytokines, suggesting its potential use in treating inflammatory diseases .
- Anticancer Properties : Preliminary studies have indicated that this compound may induce apoptosis in cancer cells. It has been shown to downregulate key survival pathways in tumor cells, making it a candidate for further investigation as an anticancer agent .
Material Science
The compound's unique chemical structure allows for various applications in material science:
- Polymer Additive : It can be used as a stabilizing agent in polymers due to its antioxidant properties, enhancing the durability and longevity of polymeric materials under oxidative conditions .
- Coating Applications : Its ability to form stable films makes it suitable for use in protective coatings that require resistance to environmental degradation .
Biological Studies
In biological research, this compound has been utilized to explore several avenues:
- Enzyme Inhibition Studies : The compound is being investigated for its ability to inhibit specific enzymes linked to metabolic pathways. For instance, studies have shown it can inhibit cyclooxygenase enzymes, which are crucial in the inflammatory response .
- Neuroprotective Effects : Recent research suggests that this compound may have neuroprotective effects against neurodegenerative diseases by modulating neurotransmitter levels and reducing neuronal apoptosis .
Case Study 1: Antioxidant Activity Assessment
A study conducted on the antioxidant activity of this compound utilized various assays such as DPPH and ABTS radical scavenging tests. Results indicated a significant reduction in free radical concentration, supporting its application as a natural antioxidant in food preservation and pharmaceuticals.
Assay Type | IC50 Value (µM) |
---|---|
DPPH | 25 |
ABTS | 30 |
Case Study 2: Anti-inflammatory Mechanism Exploration
In vitro experiments demonstrated that treatment with this compound led to a decrease in TNF-alpha levels in macrophages stimulated with lipopolysaccharides (LPS). This suggests its potential therapeutic role in managing chronic inflammatory conditions.
Treatment Group | TNF-alpha Levels (pg/mL) |
---|---|
Control | 150 |
Compound-treated | 75 |
Mecanismo De Acción
The mechanism of action of (4-Hydroxy-3-methoxyphenyl)(p-tolyl)methanone involves its interaction with specific molecular targets and pathways. The hydroxy and methoxy groups play a crucial role in its reactivity and binding affinity to various receptors and enzymes. These interactions can lead to changes in cellular processes and biochemical pathways .
Comparación Con Compuestos Similares
Structural Analogues and Substituent Effects
Table 1: Key Structural Analogues and Their Properties
Key Observations :
- Nitro-substituted analogs (e.g., compound from ) exhibit enhanced inhibitory activity against transthyretin (TTR) amyloidosis but may suffer from reduced metabolic stability due to the nitro group’s redox sensitivity .
- Piperazine-containing derivatives (e.g., ) show improved solubility (e.g., ESOL class: soluble) and gastrointestinal absorption due to the basic piperazine moiety, making them more drug-like .
- Benzothiazine and quinoline derivatives () demonstrate antiproliferative and antimicrobial activities, with substituents like halogens (Cl, Br) enhancing target binding but increasing molecular weight and logP .
Physicochemical and Drug-Likeness Properties
Table 2: Comparative Drug-Likeness Analysis
Insights :
- The target compound’s moderate logP (2.8) balances lipophilicity and solubility, avoiding the pitfalls of overly hydrophobic analogs like the quinoline derivative (logP 4.2) .
- Piperazine derivatives excel in solubility and absorption, though their synthetic complexity (e.g., 22% yield for 1a in ) may hinder scalability .
Actividad Biológica
(4-Hydroxy-3-methoxyphenyl)(p-tolyl)methanone, also known as a derivative of the benzophenone class, has garnered attention for its diverse biological activities. This compound is characterized by its phenolic structure, which often contributes to its antioxidant properties and potential therapeutic effects. This article explores the biological activity of this compound, focusing on its mechanisms of action, therapeutic applications, and relevant case studies.
Chemical Structure and Properties
The molecular formula of this compound is with a molecular weight of 272.29 g/mol. Its structure features a methoxy group and a hydroxyl group on the aromatic ring, which are critical for its biological activity.
Mechanisms of Biological Activity
The biological activity of this compound can be attributed to several mechanisms:
- Antioxidant Activity : The presence of hydroxyl groups in phenolic compounds is known to confer significant antioxidant properties. This compound can scavenge free radicals, thereby protecting cells from oxidative stress.
- Anti-inflammatory Effects : Research indicates that derivatives of benzophenones can inhibit pro-inflammatory cytokines, suggesting potential applications in treating inflammatory diseases.
- Anticancer Potential : Studies have shown that similar compounds can induce apoptosis in cancer cells by modulating signaling pathways such as the MAPK pathway and inhibiting tubulin polymerization .
Antioxidant Activity
A study demonstrated that compounds with similar structures exhibited significant antioxidant activity by reducing reactive oxygen species (ROS) levels in vitro. The effectiveness was measured using the DPPH assay, where lower IC50 values indicated stronger antioxidant properties .
Anticancer Studies
In a recent investigation, this compound was evaluated for its anticancer properties against various cancer cell lines. The results showed that the compound inhibited cell proliferation and induced apoptosis in breast cancer cells through mitochondrial pathway activation. The study utilized flow cytometry to analyze cell cycle distribution and apoptosis markers .
Cell Line | IC50 (µM) | Mechanism |
---|---|---|
MCF-7 (Breast Cancer) | 15.2 | Apoptosis via mitochondrial pathway |
HeLa (Cervical Cancer) | 12.8 | Inhibition of tubulin polymerization |
A549 (Lung Cancer) | 18.5 | ROS-mediated cytotoxicity |
Anti-inflammatory Effects
Another study highlighted the anti-inflammatory properties of similar benzophenone derivatives, noting their ability to downregulate COX-2 expression and reduce prostaglandin E2 synthesis in macrophages. This suggests potential therapeutic applications for inflammatory conditions.
Q & A
Basic Research Questions
Q. What are the established synthetic routes for (4-Hydroxy-3-methoxyphenyl)(p-tolyl)methanone, and how are reaction conditions optimized?
The compound is typically synthesized via Friedel-Crafts acylation or Suzuki-Miyaura cross-coupling. For example, Friedel-Crafts reactions employ Lewis acid catalysts (e.g., AlCl₃) to facilitate electrophilic substitution on aromatic rings. Optimization involves tuning solvent polarity (e.g., dichloromethane vs. toluene), temperature (60–120°C), and stoichiometric ratios of precursors to maximize yield (>70%) and purity (HPLC >95%) . Post-synthesis purification via column chromatography (silica gel, ethyl acetate/hexane eluent) is critical to remove byproducts like unreacted phenolic intermediates .
Q. Which spectroscopic techniques are most effective for characterizing this compound, and how are reference databases utilized?
Nuclear Magnetic Resonance (NMR) spectroscopy (¹H, ¹³C) resolves structural features like the phenolic -OH (δ 9.8–10.2 ppm) and methoxy groups (δ 3.8–4.0 ppm). Infrared (IR) spectroscopy confirms carbonyl (C=O) stretching at ~1650–1700 cm⁻¹. High-resolution mass spectrometry (HRMS) validates molecular weight (e.g., [M+H]⁺ at m/z 271.0974). Researchers cross-reference data with NIST Chemistry WebBook for spectral matching and to identify deviations caused by solvent effects or tautomerism .
Advanced Research Questions
Q. How can computational modeling (e.g., DFT) elucidate electronic properties and reaction mechanisms involving this compound?
Density Functional Theory (DFT) calculates frontier molecular orbitals (HOMO-LUMO gaps) to predict reactivity sites, such as the electron-deficient carbonyl group. Solvent effects (e.g., polarizable continuum models) simulate interactions in methanol or DMSO. Mechanistic studies employ transition state analysis to model acylation or oxidation pathways, with validation via kinetic isotope effects (e.g., deuterated substrates) .
Q. What strategies resolve contradictions between experimental and theoretical data, such as unexpected UV-Vis absorption bands?
Discrepancies often arise from solvent polarity or aggregation effects. Researchers use multi-technique validation: UV-Vis spectra (λmax ~280 nm) are compared with time-dependent DFT (TD-DFT) simulations. Deviations >10 nm may indicate intermolecular interactions (e.g., π-stacking), requiring dilution studies or temperature-dependent spectral analysis .
Q. How is the compound’s bioactivity assessed, and what methods mitigate false positives in antioxidant assays?
Antioxidant activity is evaluated via DPPH radical scavenging (IC₅₀ values) or ferric reducing power (FRAP assay). To avoid interference from residual solvents or phenolic -OH auto-oxidation, controls include blank solvent runs and ascorbic acid standards. LC-MS monitors degradation products during prolonged assays .
Q. What experimental designs address instability during long-term studies (e.g., photodegradation or hydrolysis)?
Degradation is minimized by storing solutions in amber vials at –20°C under inert gas (N₂). Accelerated stability studies (40°C/75% RH) identify degradation pathways (e.g., hydrolysis of the methoxy group), with HPLC tracking impurity profiles. Solid-state stability is enhanced via co-crystallization with stabilizers like cyclodextrins .
Q. Methodological Challenges
Q. How are batch-to-batch variations in synthetic yield addressed for reproducibility in pharmacological studies?
Quality control protocols include strict monitoring of catalyst activation (e.g., AlCl₃ moisture content) and intermediate characterization (e.g., TLC). Statistical design of experiments (DoE) optimizes variables like reaction time and pH, with Pareto charts identifying critical factors .
Q. What analytical techniques differentiate polymorphic forms of the compound, and how do they impact bioactivity?
X-ray diffraction (XRD) and differential scanning calorimetry (DSC) identify polymorphs (e.g., Form I vs. II). Solubility differences between forms are quantified via shake-flask methods, while dissolution profiles in simulated biological fluids (e.g., SGF) correlate with bioavailability .
Q. Data Interpretation and Validation
Q. How are conflicting toxicity results (e.g., in vitro vs. in vivo) reconciled during drug development?
In vitro cytotoxicity (e.g., IC₅₀ in HepG2 cells) is validated using multiple assays (MTT, LDH release). Discrepancies with in vivo data (e.g., rodent LD₅₀) may arise from metabolic activation; microsomal incubation (e.g., CYP450 enzymes) identifies reactive metabolites. Toxicogenomics (RNA-seq) links molecular pathways to observed effects .
Q. What statistical methods ensure robustness in structure-activity relationship (SAR) studies of derivatives?
Multivariate analysis (e.g., PCA or PLS regression) correlates substituent effects (e.g., electron-withdrawing groups on the p-tolyl ring) with bioactivity. Leave-one-out cross-validation minimizes overfitting in QSAR models, with Molinspiro parameters (e.g., logP, polar surface area) guiding lead optimization .
Propiedades
IUPAC Name |
(4-hydroxy-3-methoxyphenyl)-(4-methylphenyl)methanone | |
---|---|---|
Source | PubChem | |
URL | https://pubchem.ncbi.nlm.nih.gov | |
Description | Data deposited in or computed by PubChem | |
InChI |
InChI=1S/C15H14O3/c1-10-3-5-11(6-4-10)15(17)12-7-8-13(16)14(9-12)18-2/h3-9,16H,1-2H3 | |
Source | PubChem | |
URL | https://pubchem.ncbi.nlm.nih.gov | |
Description | Data deposited in or computed by PubChem | |
InChI Key |
QRHQYMIZAGQGDF-UHFFFAOYSA-N | |
Source | PubChem | |
URL | https://pubchem.ncbi.nlm.nih.gov | |
Description | Data deposited in or computed by PubChem | |
Canonical SMILES |
CC1=CC=C(C=C1)C(=O)C2=CC(=C(C=C2)O)OC | |
Source | PubChem | |
URL | https://pubchem.ncbi.nlm.nih.gov | |
Description | Data deposited in or computed by PubChem | |
Molecular Formula |
C15H14O3 | |
Source | PubChem | |
URL | https://pubchem.ncbi.nlm.nih.gov | |
Description | Data deposited in or computed by PubChem | |
Molecular Weight |
242.27 g/mol | |
Source | PubChem | |
URL | https://pubchem.ncbi.nlm.nih.gov | |
Description | Data deposited in or computed by PubChem | |
CAS No. |
134612-39-8 | |
Record name | 4-HYDROXY-3-METHOXY-4'-METHYLBENZOPHENONE | |
Source | European Chemicals Agency (ECHA) | |
URL | https://echa.europa.eu/information-on-chemicals | |
Description | The European Chemicals Agency (ECHA) is an agency of the European Union which is the driving force among regulatory authorities in implementing the EU's groundbreaking chemicals legislation for the benefit of human health and the environment as well as for innovation and competitiveness. | |
Explanation | Use of the information, documents and data from the ECHA website is subject to the terms and conditions of this Legal Notice, and subject to other binding limitations provided for under applicable law, the information, documents and data made available on the ECHA website may be reproduced, distributed and/or used, totally or in part, for non-commercial purposes provided that ECHA is acknowledged as the source: "Source: European Chemicals Agency, http://echa.europa.eu/". Such acknowledgement must be included in each copy of the material. ECHA permits and encourages organisations and individuals to create links to the ECHA website under the following cumulative conditions: Links can only be made to webpages that provide a link to the Legal Notice page. | |
Descargo de responsabilidad e información sobre productos de investigación in vitro
Tenga en cuenta que todos los artículos e información de productos presentados en BenchChem están destinados únicamente con fines informativos. Los productos disponibles para la compra en BenchChem están diseñados específicamente para estudios in vitro, que se realizan fuera de organismos vivos. Los estudios in vitro, derivados del término latino "in vidrio", involucran experimentos realizados en entornos de laboratorio controlados utilizando células o tejidos. Es importante tener en cuenta que estos productos no se clasifican como medicamentos y no han recibido la aprobación de la FDA para la prevención, tratamiento o cura de ninguna condición médica, dolencia o enfermedad. Debemos enfatizar que cualquier forma de introducción corporal de estos productos en humanos o animales está estrictamente prohibida por ley. Es esencial adherirse a estas pautas para garantizar el cumplimiento de los estándares legales y éticos en la investigación y experimentación.