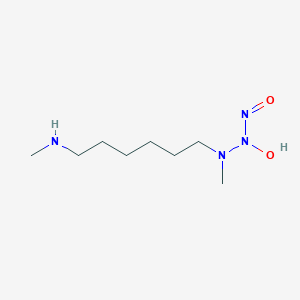
MAHMA NONOate
Descripción general
Descripción
MAHMA NONOate is a Nitric Oxide (NO) donor . It is a compound that participates in the regulation of many pathophysiological processes . It functions both as a second messenger and as an endogenous neurotransmitter . MAHMA NONOate is unstable and easy to release NO in physiological conditions .
Synthesis Analysis
MAHMA NONOate is commercially available and can be purchased from various chemical suppliers .
Molecular Structure Analysis
The molecular formula of MAHMA NONOate is C8H20N4O2 . Its average mass is 204.270 Da and its monoisotopic mass is 204.158630 Da .
Chemical Reactions Analysis
MAHMA NONOate is known to dissociate in a pH-dependent manner following first-order kinetics . It is unstable and easy to release NO in physiological conditions .
Aplicaciones Científicas De Investigación
Pharmacological Effects and Biomedical Applications
MAHMA NONOate is a type of diazeniumdiolate (NONOate), a series of compounds that are the most widely studied NO donors . They are unstable and easily release Nitric Oxide (NO) in physiological conditions . The biomedical applications and drug development of NO donors have attracted significant attention in recent years . They have a broad array of reproducible NO generation rates .
Regulation of Pathophysiological Processes
NO is a simple structured and unstable free radical molecule, which participates in the regulation of many pathophysiological processes . It functions both as a second messenger and as an endogenous neurotransmitter . Depletion of NO or attenuation of its effector system may result in hypertension, angina, and impotence . Overproduction of NO may cause damages such as circulatory shock, sepsis, neurodegenerative disorders, and inflammatory responses .
Biofilm Dispersal
MAHMA NONOate has been shown to dislodge biofilms formed by Salmonella enterica and Escherichia coli O157:H7 . Biofilms in the industrial environment can be problematic as pathogens within biofilms are significantly more resistant to chlorine and other disinfectants . MAHMA NONOate and other nitric oxide donors can induce significant dispersal of biofilms .
Potential Therapeutic Drug
NO has unique pharmacokinetics as a potential therapeutic drug . It is small, gaseous, and uncharged, and thus it can freely diffuse into tissues . However, the effects of NO are restricted locally due to its short half-life (approximately 1s) .
Inhibition of Enzyme Activities
MAHMA NONOate has been used to demonstrate that NO inhibits the activities of tobacco catalase and ascorbate peroxidase (APX) . This inhibition is reversible because removal of the NO donor led to a significant recovery .
Development of Exogenous NO Donor
Due to the multiple roles of NO in pathophysiology, intense interest in the development of exogenous NO donor has been aroused . By modifying some functional groups in the molecule, different NO-donor compounds have been designed . NONOate is one of the most extensively studied NO-donor compounds .
Mecanismo De Acción
Target of Action
MAHMA NONOate is a nitric oxide (NO) donor . Its primary targets are platelets and pulmonary arteries . Nitric oxide is a simple structured and unstable free radical molecule, which participates in the regulation of many pathophysiological processes . It functions both as a second messenger and as an endogenous neurotransmitter .
Mode of Action
MAHMA NONOate interacts with its targets by releasing nitric oxide . It has been shown to inhibit platelet aggregation induced by either collagen or ADP . This inhibitory effect was largely resistant to ODQ, a soluble guanylate cyclase inhibitor . The effect of MAHMA NONOate on platelet inhibition was approximately 10-fold more potent than S-nitrosoglutathione .
Biochemical Pathways
MAHMA NONOate affects the biochemical pathway involving the sarco-endoplasmic reticulum calcium ATPase . By activating this ATPase, it inhibits platelet aggregation . This suggests that MAHMA NONOate may play a role in the regulation of calcium homeostasis in platelets.
Pharmacokinetics
It is known that nitric oxide, which mahma nonoate releases, is small, gaseous, and uncharged, allowing it to freely diffuse into tissues . The effects of nitric oxide are restricted locally due to its short half-life (approximately 1s) .
Result of Action
The molecular and cellular effects of MAHMA NONOate’s action primarily involve the inhibition of platelet aggregation . It shows inhibitory effects to pulmonary artery and platelet aggregation with log IC 50 values of 7.18 and 6.16, respectively .
Action Environment
It is known that nitric oxide donors can be affected by light exposure
Safety and Hazards
Direcciones Futuras
The biomedical applications and drug development of NO donors like MAHMA NONOate have attracted scientists’ attention in recent years . The effect of MAHMA NONOate on platelet inhibition was approximately 10-fold more potent than S-nitrosoglutathione (GSNO). It could also reduce systemic arterial pressure more than GSNO . Therefore, in vivo, MAHMA NONOate had platelet inhibition and vasodepressor effects . This suggests that MAHMA NONOate could be of interest for clinical situations requiring transient and subtotal inhibition of platelet function .
Propiedades
IUPAC Name |
(Z)-hydroxyimino-[methyl-[6-(methylamino)hexyl]amino]-oxidoazanium | |
---|---|---|
Source | PubChem | |
URL | https://pubchem.ncbi.nlm.nih.gov | |
Description | Data deposited in or computed by PubChem | |
InChI |
InChI=1S/C8H20N4O2/c1-9-7-5-3-4-6-8-11(2)12(14)10-13/h9,13H,3-8H2,1-2H3/b12-10- | |
Source | PubChem | |
URL | https://pubchem.ncbi.nlm.nih.gov | |
Description | Data deposited in or computed by PubChem | |
InChI Key |
KQHAZGURWZYZJG-BENRWUELSA-N | |
Source | PubChem | |
URL | https://pubchem.ncbi.nlm.nih.gov | |
Description | Data deposited in or computed by PubChem | |
Canonical SMILES |
CNCCCCCCN(C)[N+](=NO)[O-] | |
Source | PubChem | |
URL | https://pubchem.ncbi.nlm.nih.gov | |
Description | Data deposited in or computed by PubChem | |
Isomeric SMILES |
CNCCCCCCN(C)/[N+](=N/O)/[O-] | |
Source | PubChem | |
URL | https://pubchem.ncbi.nlm.nih.gov | |
Description | Data deposited in or computed by PubChem | |
Molecular Formula |
C8H20N4O2 | |
Source | PubChem | |
URL | https://pubchem.ncbi.nlm.nih.gov | |
Description | Data deposited in or computed by PubChem | |
DSSTOX Substance ID |
DTXSID60163463 | |
Record name | NOC 9 | |
Source | EPA DSSTox | |
URL | https://comptox.epa.gov/dashboard/DTXSID60163463 | |
Description | DSSTox provides a high quality public chemistry resource for supporting improved predictive toxicology. | |
Molecular Weight |
204.27 g/mol | |
Source | PubChem | |
URL | https://pubchem.ncbi.nlm.nih.gov | |
Description | Data deposited in or computed by PubChem | |
Product Name |
MAHMA NONOate | |
CAS RN |
146724-86-9 | |
Record name | NOC 9 | |
Source | ChemIDplus | |
URL | https://pubchem.ncbi.nlm.nih.gov/substance/?source=chemidplus&sourceid=0146724869 | |
Description | ChemIDplus is a free, web search system that provides access to the structure and nomenclature authority files used for the identification of chemical substances cited in National Library of Medicine (NLM) databases, including the TOXNET system. | |
Record name | NOC 9 | |
Source | EPA DSSTox | |
URL | https://comptox.epa.gov/dashboard/DTXSID60163463 | |
Description | DSSTox provides a high quality public chemistry resource for supporting improved predictive toxicology. | |
Retrosynthesis Analysis
AI-Powered Synthesis Planning: Our tool employs the Template_relevance Pistachio, Template_relevance Bkms_metabolic, Template_relevance Pistachio_ringbreaker, Template_relevance Reaxys, Template_relevance Reaxys_biocatalysis model, leveraging a vast database of chemical reactions to predict feasible synthetic routes.
One-Step Synthesis Focus: Specifically designed for one-step synthesis, it provides concise and direct routes for your target compounds, streamlining the synthesis process.
Accurate Predictions: Utilizing the extensive PISTACHIO, BKMS_METABOLIC, PISTACHIO_RINGBREAKER, REAXYS, REAXYS_BIOCATALYSIS database, our tool offers high-accuracy predictions, reflecting the latest in chemical research and data.
Strategy Settings
Precursor scoring | Relevance Heuristic |
---|---|
Min. plausibility | 0.01 |
Model | Template_relevance |
Template Set | Pistachio/Bkms_metabolic/Pistachio_ringbreaker/Reaxys/Reaxys_biocatalysis |
Top-N result to add to graph | 6 |
Feasible Synthetic Routes
Q & A
Q1: How does MAHMA NONOate release nitric oxide?
A1: MAHMA NONOate spontaneously decomposes in aqueous solutions at physiological pH to release two molecules of nitric oxide (NO). This decomposition is independent of enzymatic activity. [, , ]
Q2: What are the primary downstream effects of nitric oxide released by MAHMA NONOate?
A2: Nitric oxide released from MAHMA NONOate activates soluble guanylate cyclase, leading to increased intracellular cyclic guanosine monophosphate (cGMP) levels. [, ] This increase in cGMP then triggers various downstream effects, including vasodilation, inhibition of platelet aggregation, and modulation of ion channel activity. [, , , ]
Q3: What is the molecular formula and weight of MAHMA NONOate?
A3: The molecular formula of MAHMA NONOate is C8H19N5O4, and its molecular weight is 249.26 g/mol. []
Q4: Is there any spectroscopic data available for MAHMA NONOate?
A4: Yes, MAHMA NONOate has been characterized using various spectroscopic techniques, including electrospray ionization mass spectrometry (ESI-MS). [] ESI-MS analysis has shown that MAHMA NONOate readily ionizes, releasing nitric oxide and other nitrogen oxide species upon collisional activation. []
Q5: How stable is MAHMA NONOate in solution?
A5: MAHMA NONOate's stability in solution is dependent on factors like pH and temperature. At physiological pH and 37°C, it has a half-life of approximately 15 minutes. [, ]
Q6: Are there specific storage recommendations for MAHMA NONOate?
A6: Yes, MAHMA NONOate should be stored as a dry powder at -20°C and protected from light and moisture to ensure its stability. [, ]
Q7: Does MAHMA NONOate itself possess catalytic properties?
A7: MAHMA NONOate is not inherently catalytic. Its primary function is to act as a nitric oxide donor, and its decomposition to release NO is spontaneous rather than catalytic. [, ]
Q8: What are some specific research applications of MAHMA NONOate?
A8: MAHMA NONOate is widely used in research to investigate:
- The physiological and pathological roles of nitric oxide [, , , , , ]
- Vascular reactivity and blood pressure regulation [, , , ]
- The impact of nitric oxide on platelet function [, ]
- The effects of nitric oxide on bacterial biofilms [, , ]
Q9: How do structural modifications of NONOates influence their nitric oxide release properties?
A9: The rate and duration of nitric oxide release from NONOates can be altered by modifying the amine moiety attached to the diazeniumdiolate group. [] For instance, increasing the size or complexity of the amine generally leads to a slower rate of NO release. []
Q10: What strategies can be employed to improve the stability of MAHMA NONOate in formulations?
A10: Formulating MAHMA NONOate in a dry powder form and storing it at low temperatures (-20°C) and protected from light and moisture can significantly enhance its stability. [, ]
Q11: How is MAHMA NONOate metabolized in vivo?
A11: While detailed metabolic pathways for MAHMA NONOate have not been extensively characterized, it is understood that the released nitric oxide readily interacts with various biological targets, including soluble guanylate cyclase and heme-containing enzymes. [, ] The ultimate fate of NO likely involves its oxidation to nitrate and nitrite. []
Q12: What in vitro models have been used to study the effects of MAHMA NONOate?
A12: MAHMA NONOate has been extensively used in various in vitro models, including:
- Isolated blood vessels to assess vasodilation [, , , , ]
- Platelet-rich plasma to investigate platelet aggregation inhibition [, ]
- Bacterial cultures to examine biofilm dispersal [, , ]
Q13: Has MAHMA NONOate been evaluated in animal models?
A13: Yes, MAHMA NONOate has been utilized in animal models to study its effects on:
- Blood pressure regulation in rats [, , , ]
- Cerebral blood flow in rats [, ]
- Renal function in rats []
- Erectile function in rats []
Q14: What is known about the potential toxicity of MAHMA NONOate?
A14: While MAHMA NONOate is a valuable research tool, it's essential to handle it with care. As a potent nitric oxide donor, excessive exposure could lead to undesirable effects associated with NO overproduction, such as hypotension. [, , ]
Q15: What are some alternatives to MAHMA NONOate as nitric oxide donors?
A15: Several other compounds are available that can release nitric oxide, each with its release kinetics and potential applications. Some examples include:
Descargo de responsabilidad e información sobre productos de investigación in vitro
Tenga en cuenta que todos los artículos e información de productos presentados en BenchChem están destinados únicamente con fines informativos. Los productos disponibles para la compra en BenchChem están diseñados específicamente para estudios in vitro, que se realizan fuera de organismos vivos. Los estudios in vitro, derivados del término latino "in vidrio", involucran experimentos realizados en entornos de laboratorio controlados utilizando células o tejidos. Es importante tener en cuenta que estos productos no se clasifican como medicamentos y no han recibido la aprobación de la FDA para la prevención, tratamiento o cura de ninguna condición médica, dolencia o enfermedad. Debemos enfatizar que cualquier forma de introducción corporal de estos productos en humanos o animales está estrictamente prohibida por ley. Es esencial adherirse a estas pautas para garantizar el cumplimiento de los estándares legales y éticos en la investigación y experimentación.