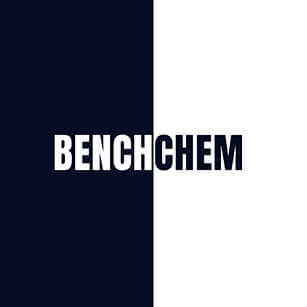
3'-O-(2-Methoxyethyl)adenosine
- Haga clic en CONSULTA RÁPIDA para recibir una cotización de nuestro equipo de expertos.
- Con productos de calidad a un precio COMPETITIVO, puede centrarse más en su investigación.
Descripción general
Descripción
3’-O-(2-Methoxyethyl)adenosine is an adenosine analogue, which means it is structurally similar to adenosine, a nucleoside that plays a crucial role in various biochemical processes. Adenosine analogues are often used in scientific research due to their ability to mimic the biological activity of adenosine.
Métodos De Preparación
Synthetic Routes and Reaction Conditions
The synthesis of 3’-O-(2-Methoxyethyl)adenosine involves multiple steps. One common method includes the following steps :
Starting Materials: (2R,3R,4R,5R)-5-(6-amino-9H-purin-9-yl)-4-(benzoyloxy)-3-(2-methoxyethoxy)tetrahydrofuran-2-yl)methyl benzoate and adenine.
Reaction Conditions: The reaction is carried out in a mixture of acetonitrile and 1,2-dichloroethane at a temperature of 10°C. Tin tetrachloride is added dropwise, and the reaction mixture is stirred at 25°C.
Purification: The reaction mixture is quenched with water, diluted with methylene chloride, and washed with water. The crude product is then dissolved in methanol/aqueous ammonia and stirred overnight at 25°C. The final product is obtained with a purity of 99.6% and a yield of 70%.
Industrial Production Methods
Industrial production methods for 3’-O-(2-Methoxyethyl)adenosine are similar to laboratory synthesis but are scaled up to accommodate larger quantities. These methods often involve optimized reaction conditions and purification techniques to ensure high yield and purity.
Análisis De Reacciones Químicas
Types of Reactions
3’-O-(2-Methoxyethyl)adenosine undergoes various chemical reactions, including:
Oxidation: This compound can be oxidized under specific conditions to form different oxidation products.
Reduction: Reduction reactions can be performed to modify the functional groups present in the compound.
Substitution: Nucleophilic substitution reactions can be carried out to replace specific groups within the molecule.
Common Reagents and Conditions
Oxidation: Common oxidizing agents include potassium permanganate and hydrogen peroxide.
Reduction: Reducing agents such as sodium borohydride and lithium aluminum hydride are used.
Substitution: Reagents like sodium hydride and lithium bromide in dimethyl sulfoxide are commonly used for substitution reactions.
Major Products Formed
The major products formed from these reactions depend on the specific conditions and reagents used. For example, oxidation may yield various oxidized derivatives, while substitution reactions can produce a range of substituted analogues.
Aplicaciones Científicas De Investigación
3’-O-(2-Methoxyethyl)adenosine has a wide range of scientific research applications, including:
Chemistry: Used as a building block in the synthesis of more complex molecules.
Biology: Studied for its role in cellular processes and its effects on smooth muscle cells.
Medicine: Investigated for its potential as a vasodilator and its ability to inhibit cancer progression.
Industry: Utilized in the development of pharmaceuticals and other biologically active compounds.
Mecanismo De Acción
The mechanism of action of 3’-O-(2-Methoxyethyl)adenosine involves its interaction with adenosine receptors in the body. By mimicking the structure of adenosine, it can bind to these receptors and exert similar biological effects. This includes the dilation of smooth muscle cells and the inhibition of cancer cell proliferation. The molecular targets and pathways involved include the activation of adenosine receptors and subsequent signaling cascades that regulate cellular functions .
Comparación Con Compuestos Similares
Similar Compounds
- Adenosine phosphate
- Acadesine
- Clofarabine
- Fludarabine phosphate
- Vidarabine
Comparison
Compared to other adenosine analogues, 3’-O-(2-Methoxyethyl)adenosine is unique due to its specific structural modifications, which enhance its biological activity and stability. For instance, the addition of the 2-methoxyethyl group increases its resistance to enzymatic degradation, making it more effective in biological systems .
Q & A
Q. Basic: What synthetic methodologies are most effective for producing 3'-O-(2-Methoxyethyl)adenosine, and how do reaction conditions influence purity?
Answer:
The synthesis of this compound typically employs phosphoramidite chemistry or chemo-enzymatic approaches . For example:
- Phosphoramidite-based synthesis involves introducing the 2-methoxyethyl group via modified nucleoside phosphoramidites, as demonstrated in the synthesis of analogous 2'-O-(2-methoxyethyl) thymidine derivatives (e.g., Scheme 1 in ) . This method allows precise control over regioselectivity but requires anhydrous conditions to avoid hydrolysis.
- Chemo-enzymatic routes use enzymes like phosphodiesterases to catalyze modifications, improving stereochemical fidelity ().
Critical factors for purity :
- Deprotection efficiency : Incomplete removal of protecting groups (e.g., dimethoxytrityl) can reduce purity. HPLC monitoring is essential .
- Solvent choice : Polar aprotic solvents (e.g., acetonitrile) minimize side reactions during coupling steps .
Table 1: Comparison of Synthetic Routes
Method | Yield (%) | Purity (%) | Key Reference |
---|---|---|---|
Phosphoramidite | 70–85 | >95 | |
Chemo-enzymatic | 60–75 | >90 |
Q. Advanced: How does the 2-methoxyethyl modification alter the metabolic stability of adenosine analogs in biological systems?
Answer:
The 2-methoxyethyl (MOE) group enhances metabolic stability by:
Steric hindrance : Blocking nuclease access to the ribose-phosphate backbone, as seen in nusinersen ().
Reduced enzymatic recognition : MOE-modified nucleosides resist degradation by adenosine deaminase, extending half-life in plasma ().
Key findings :
- In vivo stability : MOE-modified antisense oligonucleotides (ASOs) exhibit 5–10× longer half-lives compared to unmodified analogs ( ) .
- Stereochemical impact : (R)-5′-methyl-MOE derivatives show 5× higher potency than (S)-isomers in mRNA silencing ( ) .
Methodological validation :
- Use radiolabeled analogs (e.g., ³H or ¹⁴C) to track metabolic fate in hepatocyte assays .
- LC-MS/MS quantifies degradation products in serum.
Q. Basic: What analytical techniques are essential for characterizing this compound and its derivatives?
Answer:
- NMR spectroscopy : ¹H/¹³C NMR confirms regioselectivity of MOE substitution (e.g., δ 3.5–3.7 ppm for methoxy protons) .
- HPLC/UPLC : Reverse-phase chromatography (C18 columns) resolves impurities; typical mobile phase: 0.1% TFA in water/acetonitrile .
- Mass spectrometry : High-resolution MS (HRMS) validates molecular weight (e.g., [M+H]⁺ for C₁₃H₁₉N₅O₅: m/z 326.146).
Quality control protocols :
- Purity thresholds : >95% for in vitro assays; >99% for in vivo studies .
- Batch-to-batch consistency : Use qNMR with internal standards (e.g., maleic acid).
Q. Advanced: How can researchers resolve contradictions in activity data between structurally similar MOE-modified adenosine analogs?
Answer:
Discrepancies often arise from:
Stereochemical variations : (R)- vs. (S)-configured MOE groups alter target binding ( ) .
Backbone modifications : Phosphorothioate linkages (e.g., in nusinersen) enhance protein binding but may induce off-target effects.
Strategies for resolution :
- Structure-activity relationship (SAR) studies : Systematically vary MOE position and backbone chemistry ( ) .
- Crystallography : Resolve binding modes with targets (e.g., SMN2 RNA in ).
- Dose-response profiling : Compare IC₅₀ values across analogs using qRT-PCR or luciferase reporter assays .
Q. Advanced: What strategies improve the in vivo delivery and target engagement of MOE-modified adenosine therapeutics?
Answer:
- Conjugation to lipid nanoparticles (LNPs) : Enhances cellular uptake; e.g., LNPs with ionizable cationic lipids improve ASO delivery to hepatocytes .
- Peptide-linked carriers : Cell-penetrating peptides (CPPs) bypass endosomal entrapment .
- Tissue-specific targeting : GalNAc conjugates direct MOE-ASOs to hepatocytes ( ) .
Table 2: Delivery Optimization Parameters
Parameter | Optimal Range | Impact on Efficacy |
---|---|---|
Particle size | 80–120 nm | Enhanced tissue penetration |
Zeta potential | +10 to +30 mV | Improved cellular uptake |
Loading efficiency | >90% | Reduced off-target effects |
Q. Basic: What are the key structural determinants of this compound in modulating RNA interactions?
Answer:
- Ribose conformation : MOE groups lock the ribose in a C3'-endo conformation, enhancing RNA duplex stability ( ) .
- Hydrophobicity : MOE increases logP by ~0.5 units, improving membrane permeability ().
- Base pairing : MOE modifications at the 3′ position minimally disrupt Watson-Crick pairing ( ) .
Validation methods :
Propiedades
Número CAS |
303197-30-0 |
---|---|
Fórmula molecular |
C₁₃H₁₉N₅O₅ |
Peso molecular |
325.32 |
Origen del producto |
United States |
Descargo de responsabilidad e información sobre productos de investigación in vitro
Tenga en cuenta que todos los artículos e información de productos presentados en BenchChem están destinados únicamente con fines informativos. Los productos disponibles para la compra en BenchChem están diseñados específicamente para estudios in vitro, que se realizan fuera de organismos vivos. Los estudios in vitro, derivados del término latino "in vidrio", involucran experimentos realizados en entornos de laboratorio controlados utilizando células o tejidos. Es importante tener en cuenta que estos productos no se clasifican como medicamentos y no han recibido la aprobación de la FDA para la prevención, tratamiento o cura de ninguna condición médica, dolencia o enfermedad. Debemos enfatizar que cualquier forma de introducción corporal de estos productos en humanos o animales está estrictamente prohibida por ley. Es esencial adherirse a estas pautas para garantizar el cumplimiento de los estándares legales y éticos en la investigación y experimentación.