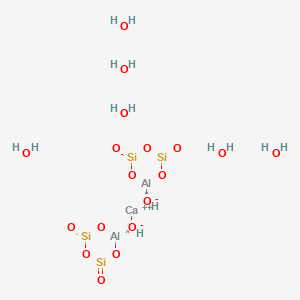
Chabazite
Descripción general
Descripción
Chabazite is a tectosilicate mineral belonging to the zeolite group. It is closely related to gmelinite and has the chemical formula (Ca,K2,Na2,Mg)Al2Si4O12•6H2O . This compound is known for its rhombohedral crystals that are pseudo-cubic in shape. The crystals can be colorless, white, orange, brown, pink, green, or yellow . It was first named “chabasie” in 1792 by Bosc d’Antic and later changed to the current spelling . This compound is commonly found in voids and amygdules in basaltic rocks .
Métodos De Preparación
Synthetic Routes and Reaction Conditions: Chabazite can be synthesized using various methods, including hydrothermal synthesis and cooperative hydration-mismatched inorganic structure-directing agents . The hydrothermal synthesis involves using hydroxide and fluoride media with organic templates such as N,N,N-Trimethyl-1-adamantammonium (TMAda) and N,N,N-Dimethylethylcyclohexyl ammonium (DMECHA) . These templates help in forming pure silica this compound without impurities . Another method involves using a ternary mixture of sodium, potassium, and cesium cations to reduce synthesis times and temperatures .
Industrial Production Methods: Industrial production of this compound often involves the use of organic-free syntheses to avoid long synthesis times and impurity formation . The use of lithium as an accelerant in small quantities has been shown to be effective in this compound crystallization . This method expands the design space of this compound formation compared to conventional methods utilizing potassium as the sole structure-directing agent .
Análisis De Reacciones Químicas
Types of Reactions: Chabazite undergoes various chemical reactions, including ion exchange, adsorption, and catalytic reactions . It is particularly known for its role in ammonia-assisted selective reduction of nitrogen oxides (NH3-SCR) in diesel exhaust treatment .
Common Reagents and Conditions: Common reagents used in this compound reactions include ammonia, copper ions, and various metal cations . The conditions for these reactions often involve low temperatures and the presence of specific cations to facilitate the desired chemical transformations .
Major Products: The major products formed from this compound reactions include reduced nitrogen oxides, ammonium nitrate, and various ion-exchanged forms of this compound .
Aplicaciones Científicas De Investigación
Chabazite has a wide range of scientific research applications, including:
Catalysis: this compound is used as a catalyst in the selective catalytic reduction of nitrogen oxides with ammonia (NH3-SCR).
Water Treatment: this compound is effective in removing radioactive cesium from contaminated water, making it valuable in nuclear waste management
Photocatalysis: Modified this compound samples have shown high efficiency in the photocatalytic degradation of methylene blue dye under ultraviolet light.
Gas Separation: this compound is used as an adsorbent in gas separation processes due to its unique pore structure.
Mecanismo De Acción
The mechanism of action of chabazite involves its open-framework structure composed of [SiO4]4- and [AlO4]5- tetrahedra . The presence of aluminum in the framework creates negatively charged sites that are balanced by exchangeable cations such as sodium, potassium, or calcium . These cations can be exchanged with other cations in solution, allowing this compound to act as an ion exchanger . In catalytic applications, the dynamic motion of solvated copper sites in this compound plays a crucial role in the reduction of nitrogen oxides .
Comparación Con Compuestos Similares
Chabazite is similar to other zeolites such as gmelinite, silicoaluminophosphate, and aluminosilicate forms like AEI and AFX . this compound is unique due to its high selectivity for cesium ion exchange and its effectiveness in low-temperature catalytic reactions . Other similar compounds include:
Gmelinite: Similar in structure but differs in its ion exchange properties.
Silicoaluminophosphate (SAPO): Used in similar catalytic applications but has different pore structures.
Aluminosilicate (AEI, AFX): These zeolites have different framework structures and catalytic properties compared to this compound.
This compound’s unique combination of ion exchange capacity, catalytic activity, and structural properties makes it a valuable material in various scientific and industrial applications.
Actividad Biológica
Chabazite, a naturally occurring zeolite, has garnered attention for its potential biological activities, particularly in the context of oxidative stress and environmental remediation. This article delves into the biological effects of this compound, highlighting its antioxidant properties, applications in wastewater treatment, and synthesis methods that enhance its efficacy.
Antioxidant Properties
Recent studies have demonstrated that this compound can significantly influence antioxidant enzyme levels in humans. A key study evaluated the impact of natural zeolites, including this compound, on oxidative stress markers in clinically healthy individuals. The results indicated that after four weeks of this compound intake, there was a notable increase in the levels of several antioxidant enzymes:
- Glutathione Peroxidase (GSH-Px)
- Superoxide Dismutase (SOD)
- Glutathione Reductase (GSH-R)
These enzymes play crucial roles in mitigating oxidative damage by neutralizing reactive oxygen species (ROS). Specifically, the study reported a decrease in thiobarbituric acid reactive substances (TBARS), which are indicators of lipid peroxidation and oxidative stress. This suggests that this compound may serve as a protective agent against oxidative damage, particularly in populations exposed to various stressors such as smoking .
Table 1: Effects of this compound on Antioxidant Enzymes
Enzyme | Baseline Level (T0) | Post-Intake Level (T1) | Change |
---|---|---|---|
Glutathione Peroxidase | Lower in smokers | Increased | Significant increase |
Superoxide Dismutase | Lower in smokers | Increased | Significant increase |
Glutathione Reductase | Lower in smokers | Increased | Significant increase |
Total Antioxidant Status | Higher than normal | Decreased | Remained higher than normal |
The mechanisms underlying these antioxidant effects are not fully understood but may involve indirect interactions with biochemical systems, including toxin removal and immune system activation .
Applications in Wastewater Treatment
This compound has also been explored for its utility in environmental applications, particularly in wastewater treatment. A case study investigated the use of this compound to mitigate ammonia levels in wastewater from a marine fish farm. The results indicated that this compound effectively reduced ammonium and soluble reactive phosphorus concentrations, demonstrating its potential as a sustainable solution for nutrient removal in aquaculture settings .
Table 2: Impact of this compound on Ammonium Levels
Parameter | Before this compound Addition | After this compound Addition | Change |
---|---|---|---|
Ammonium Concentration (mg/L) | Higher than input | Decreased | Significant decrease |
Nitrate Concentration (mg/L) | Lower than input | Increased | Resumption of nitrification |
This application highlights the versatility of this compound not only as a health supplement but also as an effective agent for environmental remediation.
Synthesis and Enhancement Techniques
The synthesis of this compound has evolved to improve its performance. Recent research has focused on various methods to create high-silica this compound zeolites using different inorganic cations. These advancements allow for better control over the structural properties and enhance the zeolite's efficacy in biological applications .
Table 3: Synthesis Methods for this compound
Method | Description |
---|---|
Interzeolite Conversion | Transforming FAU-type zeolites to this compound using organic structure-directing agents. |
Seed-Free Synthesis | Developing this compound without organic agents to reduce impurities and synthesis time. |
Propiedades
InChI |
InChI=1S/2Al.Ca.2O5Si2.8H2O/c;;;2*1-6(2)5-7(3)4;;;;;;;;/h;;;;;8*1H2/q3*+2;2*-2;;;;;;;;/p-2 | |
---|---|---|
Source | PubChem | |
URL | https://pubchem.ncbi.nlm.nih.gov | |
Description | Data deposited in or computed by PubChem | |
InChI Key |
UNYSKUBLZGJSLV-UHFFFAOYSA-L | |
Source | PubChem | |
URL | https://pubchem.ncbi.nlm.nih.gov | |
Description | Data deposited in or computed by PubChem | |
Canonical SMILES |
O.O.O.O.O.O.[OH-].[OH-].O=[Si]1O[Al]O[Si](=O)O1.O=[Si]1O[Al]O[Si](=O)O1.[Ca+2] | |
Source | PubChem | |
URL | https://pubchem.ncbi.nlm.nih.gov | |
Description | Data deposited in or computed by PubChem | |
Molecular Formula |
Al2CaH14O18Si4 | |
Source | PubChem | |
URL | https://pubchem.ncbi.nlm.nih.gov | |
Description | Data deposited in or computed by PubChem | |
DSSTOX Substance ID |
DTXSID101019922 | |
Record name | Chabazite | |
Source | EPA DSSTox | |
URL | https://comptox.epa.gov/dashboard/DTXSID101019922 | |
Description | DSSTox provides a high quality public chemistry resource for supporting improved predictive toxicology. | |
Molecular Weight |
508.48 g/mol | |
Source | PubChem | |
URL | https://pubchem.ncbi.nlm.nih.gov | |
Description | Data deposited in or computed by PubChem | |
CAS No. |
12251-32-0 | |
Record name | Chabazite | |
Source | ChemIDplus | |
URL | https://pubchem.ncbi.nlm.nih.gov/substance/?source=chemidplus&sourceid=0012251320 | |
Description | ChemIDplus is a free, web search system that provides access to the structure and nomenclature authority files used for the identification of chemical substances cited in National Library of Medicine (NLM) databases, including the TOXNET system. | |
Record name | Chabazite | |
Source | EPA DSSTox | |
URL | https://comptox.epa.gov/dashboard/DTXSID101019922 | |
Description | DSSTox provides a high quality public chemistry resource for supporting improved predictive toxicology. | |
Q1: What is Chabazite?
A: this compound is a naturally occurring zeolite, a family of microporous aluminosilicate minerals. [, , ] It's known for its unique structure featuring large ellipsoidal cavities accessed through eight-membered rings, making it a promising candidate for various applications. [, ]
Q2: How does the cation exchange capacity of this compound compare to other zeolites?
A: this compound, particularly its synthetic high-aluminum variant (Al-chab), possesses a high ion-exchange capacity, potentially reaching the theoretical zeolite limit. [] This higher capacity is attributed to the significant aluminum substitution for silicon in its framework. [] Compared to clinoptilolite, another natural zeolite, this compound exhibits a superior exchange capacity due to its higher aluminum content, making its framework more negatively charged and thus favorable for ion exchange. []
Q3: What are the common techniques used to characterize this compound?
A: Characterizing this compound involves various techniques like X-ray diffraction (XRD) to determine its crystalline structure, Fourier transform infrared spectroscopy (FTIR) to identify its functional groups, and N2 adsorption/desorption studies to evaluate surface area and pore size distribution. [, , , , , ] Researchers also employ electron microprobe analysis to determine its elemental composition. [, ]
Q4: How does the method used for porosity characterization affect the results obtained for this compound?
A: Traditional methods of porosity characterization, like nitrogen adsorption at 77 K, may not be suitable for chabazites, particularly those with large cations like potassium. [] These large cations can block the access of nitrogen molecules to the pore space, leading to an underestimation of surface area and pore volume. Using carbon dioxide at 273 K, a smaller molecule, provides more accurate results, revealing the true porosity of such chabazites. []
Q5: How do different cations affect the adsorption behavior of carbon dioxide in this compound?
A: The type of cation present in this compound influences carbon dioxide's adsorption behavior. While carbon dioxide generally exhibits high affinity within this compound pores, studies on sodium (NaCHA) and potassium (KCHA) exchanged chabazites revealed anomalous behavior at low pressures. [] This suggests steric hindrance and potential pore blockage for carbon dioxide molecules, particularly in KCHA due to the larger potassium cation size. []
Q6: What is the significance of studying the low-pressure adsorption behavior of gases in this compound?
A: Investigating the low-pressure adsorption behavior provides insights into the fundamental adsorbate-adsorbent interactions. [] Analyzing data in the Henry's law region helps understand the initial stages of adsorption and the nature of interactions between gas molecules and the this compound framework. []
Q7: What causes hysteresis in the carbon dioxide desorption isotherms of this compound?
A: Low-pressure hysteresis observed in carbon dioxide desorption isotherms at 273 K, particularly for NaCHA and KCHA, suggests pore blockage and encapsulation of carbon dioxide molecules within the this compound structure. [] This phenomenon might be attributed to the strong interaction between carbon dioxide and the cation-exchanged framework, hindering its release at low pressures. []
Q8: How does the choice of computational model affect the accuracy of predicting carbon dioxide encapsulation in this compound?
A: Accurately modeling the encapsulation process in this compound requires carefully considering the adsorbate-adsorbate and adsorbate-host interactions. [] Studies show that solely relying on the radial distribution function (rdf) and 3D lattice gas theory underestimates the number of encapsulated molecules. [] Incorporating Lennard-Jones and quadrupolar potentials to describe these interactions improves the model's predictive capabilities. []
Q9: What insights have computational studies provided regarding copper(II) cation location and behavior in this compound?
A: Electron spin resonance and electron spin echo modulation studies, combined with computational modeling, revealed that in hydrated this compound, copper(II) ions are likely located above the six-membered ring, slightly displaced into the ellipsoidal cavity. [] This location allows coordination with three water molecules and three framework oxygens. [] Upon dehydration, two distinct copper(II) species emerge, indicating a shift in coordination environment. []
Q10: How does the interaction of copper(II) with adsorbates in this compound compare to SAPO-34?
A: While copper(II) in both this compound and its silicoaluminophosphate analogue, SAPO-34, interacts with adsorbates, differences exist. [] For instance, in dehydrated this compound, only copper(II) located in the hexagonal rings interacts with ethylene, whereas copper(II) in both hydrated and dehydrated SAPO-34 readily complexes with ethylene. [] These variations are attributed to the distinct cation densities and acidic properties of these materials. [, ]
Q11: What makes this compound a potential material for environmental remediation?
A: this compound's high ion-exchange capacity and large surface area make it suitable for removing heavy metals from contaminated water. [, , ] Studies demonstrate its effectiveness in adsorbing lead, cadmium, copper, zinc, chromium, nickel, and cobalt. [, , ] Its performance is influenced by factors like metal concentration, pH, and the presence of competing ions. [, ]
Q12: How effective is this compound in removing methylene blue dye from aqueous solutions?
A: Research indicates that natural and synthesized this compound efficiently removes methylene blue dye from aqueous solutions. [, ] The adsorption process follows the Langmuir model and pseudo-second-order kinetics. [] The maximum adsorption capacity of this compound for methylene blue is significantly higher than that of the raw materials used for its synthesis, highlighting its effectiveness as an adsorbent. []
Q13: What is the role of this compound in the bioremediation of lead toxicity in fish?
A: this compound's ability to adsorb lead makes it a potential tool for mitigating lead toxicity in fish. Studies on Heteropneustes fossilis exposed to lead nitrate show that adding this compound to the water improves protein content in various tissues (brain, liver, kidney, gills) compared to lead-exposed fish without this compound. [] This improvement suggests this compound's potential in reducing lead bioavailability and toxicity in aquatic environments. []
Q14: How does this compound contribute to nitrogen removal in wastewater treatment?
A: this compound plays a crucial role in enhancing nitrification, a key step in nitrogen removal from wastewater, especially for treating high-strength wastewaters like anaerobically digested livestock waste centrate. [, ] The zeolite's high affinity for ammonium ions allows it to act as a temporary storage reservoir, reducing free ammonia (FA) concentrations that inhibit nitrification. [, ] Subsequent bioregeneration of this compound via nitrification allows for sustained nitrogen removal. []
Q15: How effective are this compound-amended sequencing batch reactors (SBRs) in removing nitrogen from wastewater?
A: Studies demonstrate that incorporating this compound into SBRs significantly enhances nitrogen removal from anaerobically digested swine waste centrate. [] By mitigating FA inhibition through ammonium adsorption, this compound-amended SBRs achieve high total nitrogen removal efficiencies (up to 84%). [] This highlights the potential of integrating zeolites into existing wastewater treatment systems for improved nitrogen removal. []
Q16: How does the application of this compound affect nitrogen dynamics in soil?
A: Incorporating natural and NH4+-enriched this compound into soil impacts nitrogen dynamics, particularly ammonification and nitrification rates. [, ] While natural this compound shows a modest effect, primarily delaying nitrate production, NH4+-enriched this compound stimulates NH4+-N production and N2O-N emissions while reducing NO3−-N production. [] This difference emphasizes the impact of zeolite modification on its influence on soil nitrogen cycling. [, ]
Q17: Can this compound be used in conjunction with nitrification inhibitors?
A: Research suggests compatibility between this compound (both natural and NH4+-enriched) and the nitrification inhibitor DMPP (3,4-dimethylpyrazole phosphate). [] Using NH4+-enriched this compound with DMPP can help mitigate short-term nitrogen losses from the soil while providing a sustainable method for nutrient recycling. []
Q18: How does this compound perform in acidic sandy soil compared to other nitrogen sources?
A: Studies comparing NH4+-enriched this compound with urea and composted manure in acidic sandy-loam soil reveal distinct behaviors. [] NH4+-enriched zeolite displays a balanced profile for net NO3−-N production and NH4+-N consumption compared to the slow N mineralization of manure and the temporary pH changes induced by urea. [] This suggests that N-enriched this compound is a promising alternative N source for acidic soils, promoting balanced nutrient availability. []
Q19: How does this compound interact with Plant Growth Promoting Rhizobacteria (PGPR) to affect plant growth?
A: this compound, especially when enriched with PGPR, demonstrates the potential to optimize fertilizer use and enhance plant growth. [, ] Studies on Ranunculus asiaticus and Opuntia ficus-indica indicate that this compound combined with PGPR improves agronomic parameters, photosynthetic rate, chlorophyll content, and mineral uptake compared to plants grown without this compound or with sterilized this compound. [, ] This suggests a synergistic effect between this compound and beneficial microorganisms, potentially related to improved nutrient and water absorption by plant roots. [, ]
Q20: Can this compound be used to enhance plant protection against diseases?
A: Research shows that micronized this compound, particularly when enriched with microorganisms, effectively protects plants against fungal diseases. [, ] Studies on Vitis vinifera demonstrate its efficacy in controlling Botrytis cinerea, Oidium tuckeri, and downy mildew, even surpassing the performance of traditional copper and sulfur treatments. [] Similarly, this compound shows promise in safeguarding tomato plants against Phytophthora infestans and Leivellula taurica. [] This protective effect is attributed to the zeolite's ability to create a physical barrier against pathogens and its interaction with microorganisms that can enhance plant defense mechanisms. [, ]
Q21: How does this compound contribute to the immobilization of radioactive cesium?
A: this compound plays a vital role in immobilizing radioactive cesium, a significant concern in nuclear waste management. [, ] Studies show that incorporating this compound into cementitious mixes used for immobilizing radioactive waste effectively traps cesium within its structure. [] The formation of this compound during the hydration of these mixes results in the stable retention of cesium, even after exposure to high temperatures (up to 750°C). [, ] This stability is attributed to the strong ceramic bonds within the zeolite structure. []
Q22: What are the advantages of using this compound for cesium immobilization compared to other methods?
A: Using this compound for cesium immobilization offers several advantages. Firstly, its high selectivity for cesium ensures efficient removal from complex waste streams. [] Secondly, the immobilization process occurs at relatively low temperatures (50°C), making it energy-efficient. [] Finally, the thermal stability of this compound ensures long-term containment of the radioactive cesium, even after exposure to high temperatures during potential storage or disposal scenarios. []
Q23: Can this compound be synthesized from waste materials?
A: Yes, this compound can be synthesized from various waste materials, promoting sustainability and resource recycling. [, , ] Successfully synthesized from construction waste and kaolin, the resulting this compound exhibits comparable properties to natural this compound, including its ability to adsorb pollutants like methylene blue and carbon dioxide. [, , ] This highlights the potential of utilizing industrial by-products for synthesizing valuable zeolitic materials. [, , ]
Q24: How does the silica content of this compound affect its properties and applications?
A: The silica content significantly impacts this compound's properties and suitability for specific applications. High silica this compound, like H-SSZ-13, exhibits strong Brønsted acidity, making it a potential catalyst for reactions like methanol-to-olefins conversion. [] Conversely, low silica this compound, often found in specific geological formations, may exhibit distinct ion exchange and adsorption behaviors compared to their high silica counterparts. []
Q25: What is the significance of platy morphology in this compound?
A: The platy morphology of certain this compound types, such as the sedimentary variety, provides a large external surface area accessible to larger molecules. [] This is particularly advantageous for applications involving bitumen cracking and upgrading, where the accessible surface area facilitates interaction with large hydrocarbon molecules like asphaltenes. []
Q26: How can this compound be used in the dehydration of natural gas?
A: Synthesized this compound membranes demonstrate potential for dehydrating natural gas due to their selective permeation properties. [] These membranes effectively separate water vapor from methane, achieving high ideal selectivities. [] Optimizing synthesis parameters, such as temperature, is crucial for maximizing the separation performance of these membranes. []
Q27: What makes this compound a suitable material for supporting silver nanoparticles?
A: Both natural and synthetic this compound exhibit the unique ability to template the formation of silver nanoparticles on their surfaces. [, ] This is attributed to the specific surface chemistry and morphology of this compound, which facilitates the nucleation and growth of silver nanoparticles with controlled size and distribution. [, ] These silver-supported this compound materials hold promise for various applications, including catalysis and antibacterial agents. []
Descargo de responsabilidad e información sobre productos de investigación in vitro
Tenga en cuenta que todos los artículos e información de productos presentados en BenchChem están destinados únicamente con fines informativos. Los productos disponibles para la compra en BenchChem están diseñados específicamente para estudios in vitro, que se realizan fuera de organismos vivos. Los estudios in vitro, derivados del término latino "in vidrio", involucran experimentos realizados en entornos de laboratorio controlados utilizando células o tejidos. Es importante tener en cuenta que estos productos no se clasifican como medicamentos y no han recibido la aprobación de la FDA para la prevención, tratamiento o cura de ninguna condición médica, dolencia o enfermedad. Debemos enfatizar que cualquier forma de introducción corporal de estos productos en humanos o animales está estrictamente prohibida por ley. Es esencial adherirse a estas pautas para garantizar el cumplimiento de los estándares legales y éticos en la investigación y experimentación.