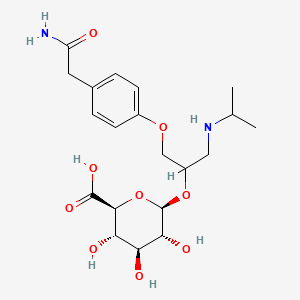
Atenolol beta-D-Glucuronide
Descripción general
Descripción
Atenolol beta-D-Glucuronide is a glucuronide conjugate of atenolol, a beta-1 selective adrenergic receptor antagonist. Atenolol is commonly used in the management of hypertension, angina pectoris, and other cardiovascular conditions. The glucuronide conjugate is formed through the process of glucuronidation, which is a major phase II metabolic pathway in the body. This conjugation enhances the solubility of atenolol, facilitating its excretion from the body.
Métodos De Preparación
Synthetic Routes and Reaction Conditions
The synthesis of Atenolol beta-D-Glucuronide involves the conjugation of atenolol with glucuronic acid. The reaction typically occurs in the liver, catalyzed by the enzyme UDP-glucuronosyltransferase. In vitro synthesis can be achieved by reacting atenolol with activated glucuronic acid derivatives under mild conditions. The reaction is usually carried out in an aqueous medium at a pH of around 7.4, with the presence of a suitable catalyst to facilitate the conjugation.
Industrial Production Methods
Industrial production of this compound involves large-scale enzymatic reactions using recombinant UDP-glucuronosyltransferase. The process is optimized to ensure high yield and purity of the product. The reaction mixture is subjected to purification steps such as chromatography to isolate the glucuronide conjugate from unreacted atenolol and other by-products.
Análisis De Reacciones Químicas
Types of Reactions
Atenolol beta-D-Glucuronide primarily undergoes hydrolysis and conjugation reactions. The glucuronide moiety can be cleaved by beta-glucuronidase enzymes, releasing the parent compound, atenolol. Additionally, the compound can participate in phase II metabolic reactions, forming further conjugates with sulfate or glutathione.
Common Reagents and Conditions
Hydrolysis: Catalyzed by beta-glucuronidase enzymes, typically occurring in the liver and intestines.
Conjugation: Involves UDP-glucuronic acid as the glucuronide donor, catalyzed by UDP-glucuronosyltransferase.
Major Products Formed
The major product formed from the hydrolysis of this compound is atenolol. Further conjugation reactions can lead to the formation of sulfate or glutathione conjugates.
Aplicaciones Científicas De Investigación
Atenolol beta-D-Glucuronide has several applications in scientific research:
Pharmacokinetics: Used to study the metabolism and excretion of atenolol in the body.
Toxicology: Helps in understanding the detoxification pathways and the role of glucuronidation in drug metabolism.
Drug Development: Serves as a model compound to investigate the effects of glucuronidation on drug solubility and excretion.
Biochemistry: Used in studies involving enzyme kinetics and the role of UDP-glucuronosyltransferase in drug metabolism.
Mecanismo De Acción
Atenolol beta-D-Glucuronide exerts its effects through the parent compound, atenolol. Atenolol is a cardioselective beta-blocker that selectively binds to beta-1 adrenergic receptors in the heart. This binding inhibits the action of catecholamines, leading to a decrease in heart rate and myocardial contractility. The glucuronide conjugate itself does not have significant pharmacological activity but serves as a means to enhance the excretion of atenolol from the body.
Comparación Con Compuestos Similares
Similar Compounds
Metoprololbeta-D-Glucuronide: Another beta-1 selective adrenergic receptor antagonist glucuronide conjugate.
Propranololbeta-D-Glucuronide: A non-selective beta-blocker glucuronide conjugate.
Bisoprololbeta-D-Glucuronide: A beta-1 selective adrenergic receptor antagonist glucuronide conjugate.
Uniqueness
Atenolol beta-D-Glucuronide is unique in its selective action on beta-1 adrenergic receptors, which makes it preferable for patients with respiratory conditions such as asthma. Its glucuronide conjugate form enhances its solubility and excretion, reducing the risk of accumulation and potential side effects.
Actividad Biológica
Atenolol beta-D-glucuronide is a metabolite of atenolol, a selective beta-1 adrenergic receptor antagonist commonly used to treat hypertension and other cardiovascular conditions. Understanding the biological activity of this glucuronide metabolite is crucial for evaluating its pharmacological effects and potential therapeutic applications.
Pharmacokinetics and Metabolism
Atenolol undergoes minimal hepatic metabolism, with about 5% converted into metabolites, including hydroxyatenolol and this compound . The glucuronidation process enhances the solubility and excretion of atenolol, primarily through renal pathways. The pharmacokinetic profile indicates that this compound may have different bioavailability and activity compared to its parent compound.
Table 1: Pharmacokinetic Properties of Atenolol and Its Metabolites
Property | Atenolol | This compound |
---|---|---|
Bioavailability | 50-60% | Unknown |
Protein Binding | 6-16% | Unknown |
Metabolism | Minimal (5%) | Glucuronidation |
Elimination Half-Life | 6-7 hours | Unknown |
Major Excretion Route | Renal (47%) | Renal (assumed) |
Biological Activity
The biological activity of this compound has not been extensively studied, but it is assumed to be less pharmacologically active than atenolol itself. The primary function of glucuronidation is to facilitate the elimination of drugs, which suggests that this metabolite may have limited therapeutic effects.
Case Studies
- Cardiovascular Effects : A study examining the pharmacodynamics of atenolol revealed that the parent compound effectively reduces heart rate and blood pressure by blocking beta-1 adrenergic receptors . However, the specific contribution of this compound to these effects remains unclear.
- Renal Excretion : Research on the renal clearance of beta-blockers indicates that metabolites like this compound are likely excreted in urine, which may influence the overall pharmacokinetics of atenolol in patients with varying renal function .
- Environmental Impact : A study on the fate of beta-blockers in wastewater treatment highlighted that glucuronides, including this compound, are more persistent in the environment than their parent compounds, raising concerns about their ecological impact .
Propiedades
IUPAC Name |
(2S,3S,4S,5R,6R)-6-[1-[4-(2-amino-2-oxoethyl)phenoxy]-3-(propan-2-ylamino)propan-2-yl]oxy-3,4,5-trihydroxyoxane-2-carboxylic acid | |
---|---|---|
Source | PubChem | |
URL | https://pubchem.ncbi.nlm.nih.gov | |
Description | Data deposited in or computed by PubChem | |
InChI |
InChI=1S/C20H30N2O9/c1-10(2)22-8-13(9-29-12-5-3-11(4-6-12)7-14(21)23)30-20-17(26)15(24)16(25)18(31-20)19(27)28/h3-6,10,13,15-18,20,22,24-26H,7-9H2,1-2H3,(H2,21,23)(H,27,28)/t13?,15-,16-,17+,18-,20+/m0/s1 | |
Source | PubChem | |
URL | https://pubchem.ncbi.nlm.nih.gov | |
Description | Data deposited in or computed by PubChem | |
InChI Key |
TUFRIQODZMVHRP-RLYWBOKJSA-N | |
Source | PubChem | |
URL | https://pubchem.ncbi.nlm.nih.gov | |
Description | Data deposited in or computed by PubChem | |
Canonical SMILES |
CC(C)NCC(COC1=CC=C(C=C1)CC(=O)N)OC2C(C(C(C(O2)C(=O)O)O)O)O | |
Source | PubChem | |
URL | https://pubchem.ncbi.nlm.nih.gov | |
Description | Data deposited in or computed by PubChem | |
Isomeric SMILES |
CC(C)NCC(COC1=CC=C(C=C1)CC(=O)N)O[C@H]2[C@@H]([C@H]([C@@H]([C@H](O2)C(=O)O)O)O)O | |
Source | PubChem | |
URL | https://pubchem.ncbi.nlm.nih.gov | |
Description | Data deposited in or computed by PubChem | |
Molecular Formula |
C20H30N2O9 | |
Source | PubChem | |
URL | https://pubchem.ncbi.nlm.nih.gov | |
Description | Data deposited in or computed by PubChem | |
DSSTOX Substance ID |
DTXSID101112968 | |
Record name | 2-[4-(2-Amino-2-oxoethyl)phenoxy]-1-[[(1-methylethyl)amino]methyl]ethyl β-D-glucopyranosiduronic acid | |
Source | EPA DSSTox | |
URL | https://comptox.epa.gov/dashboard/DTXSID101112968 | |
Description | DSSTox provides a high quality public chemistry resource for supporting improved predictive toxicology. | |
Molecular Weight |
442.5 g/mol | |
Source | PubChem | |
URL | https://pubchem.ncbi.nlm.nih.gov | |
Description | Data deposited in or computed by PubChem | |
CAS No. |
889359-84-6 | |
Record name | 2-[4-(2-Amino-2-oxoethyl)phenoxy]-1-[[(1-methylethyl)amino]methyl]ethyl β-D-glucopyranosiduronic acid | |
Source | CAS Common Chemistry | |
URL | https://commonchemistry.cas.org/detail?cas_rn=889359-84-6 | |
Description | CAS Common Chemistry is an open community resource for accessing chemical information. Nearly 500,000 chemical substances from CAS REGISTRY cover areas of community interest, including common and frequently regulated chemicals, and those relevant to high school and undergraduate chemistry classes. This chemical information, curated by our expert scientists, is provided in alignment with our mission as a division of the American Chemical Society. | |
Explanation | The data from CAS Common Chemistry is provided under a CC-BY-NC 4.0 license, unless otherwise stated. | |
Record name | 2-[4-(2-Amino-2-oxoethyl)phenoxy]-1-[[(1-methylethyl)amino]methyl]ethyl β-D-glucopyranosiduronic acid | |
Source | EPA DSSTox | |
URL | https://comptox.epa.gov/dashboard/DTXSID101112968 | |
Description | DSSTox provides a high quality public chemistry resource for supporting improved predictive toxicology. | |
Descargo de responsabilidad e información sobre productos de investigación in vitro
Tenga en cuenta que todos los artículos e información de productos presentados en BenchChem están destinados únicamente con fines informativos. Los productos disponibles para la compra en BenchChem están diseñados específicamente para estudios in vitro, que se realizan fuera de organismos vivos. Los estudios in vitro, derivados del término latino "in vidrio", involucran experimentos realizados en entornos de laboratorio controlados utilizando células o tejidos. Es importante tener en cuenta que estos productos no se clasifican como medicamentos y no han recibido la aprobación de la FDA para la prevención, tratamiento o cura de ninguna condición médica, dolencia o enfermedad. Debemos enfatizar que cualquier forma de introducción corporal de estos productos en humanos o animales está estrictamente prohibida por ley. Es esencial adherirse a estas pautas para garantizar el cumplimiento de los estándares legales y éticos en la investigación y experimentación.