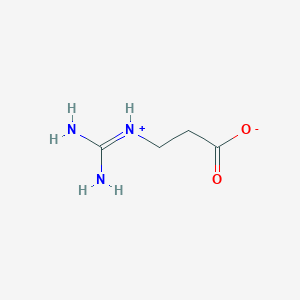
Ácido 3-Guanidinopropiónico
Descripción general
Descripción
Aplicaciones Científicas De Investigación
RGX-202 tiene varias aplicaciones de investigación científica, incluyendo:
Biología: RGX-202 se utiliza para investigar el papel del transportador de creatina SLC6A8 en el metabolismo energético celular y su impacto en varios procesos biológicos.
Medicina: RGX-202 se está explorando como un posible agente terapéutico para tratar la distrofia muscular de Duchenne y el cáncer colorrectal. .
Mecanismo De Acción
RGX-202 ejerce sus efectos inhibiendo competitivamente el transportador de creatina SLC6A8. Esta inhibición previene la absorción de fosfocreatina en las células, interrumpiendo así el metabolismo energético celular. La reducción en los niveles de fosfocreatina intracelular lleva a una disminución de la producción de ATP, que es esencial para la supervivencia y proliferación celular. Este mecanismo es particularmente efectivo en las células cancerosas que dependen de la vía SLC6A8 para la producción de energía en condiciones hipóxicas .
Análisis Bioquímico
Biochemical Properties
3-GPA has been shown to competitively inhibit creatine transport across the plasmalemma . It interacts with the creatine transporter, and other creatine analogs appear to be transported themselves by the creatine transporter . Uptake was shown to be inhibited most efficiently and in a competitive manner by 3-GPA .
Cellular Effects
3-GPA has been shown to decrease intracellular creatine and phosphocreatine in all tissues studied . In skeletal muscle, this effect induced a shift from glycolytic to oxidative metabolism, increased cellular glucose uptake, and increased fatigue tolerance . In heart tissue, this shift to mitochondrial metabolism was less pronounced .
Molecular Mechanism
3-GPA is an endogenous AMP-activated protein kinase (AMPK) stimulator and PPARγ coactivator 1α (PGC-1α) activator . AMPK is an enzyme involved in cellular energy homeostasis . PGC-1α activation results in enhanced expression of AMPK, as well as genes for oxidative phosphorylation, electron transport chain, and mitochondrial biogenesis .
Temporal Effects in Laboratory Settings
When fed to rats and mice, 3-GPA was shown to cause the concentrations of creatine and phosphocreatine in heart and skeletal muscle to progressively decrease over time . Furthermore, in animals fed 3-GPA, the accumulation of these creatine analogs within the tissues is paralleled by a decline in intracellular creatine and phosphocreatine concentrations .
Dosage Effects in Animal Models
In animal models of non-insulin-dependent diabetes mellitus (NIDDM), 3-GPA improved insulin sensitivity and promoted weight loss selectively from adipose tissue . Treatment of animals with 3-GPA has not only been shown to significantly lower body weight, but also retards growth relative to normal controls . 3-GPA will even cause young animals to stop eating and often die .
Metabolic Pathways
3-GPA may be formed by L-arginine:glycine amidinotransferase through transamidination between arginine and β-alanine . It plays a pivotal role in the storage and utilization of phosphate-bound energy via creatine kinase in the brain, thus serving to maintain energy homeostasis in the brain .
Transport and Distribution
3-GPA is transported and distributed within cells and tissues via the creatine transporter . It has been shown to competitively inhibit creatine transport across the plasmalemma .
Métodos De Preparación
Rutas de Síntesis y Condiciones de Reacción
La síntesis de RGX-202 involucra múltiples pasos, incluyendo la preparación de intermediarios y las reacciones de acoplamiento finales. Las rutas de síntesis específicas y las condiciones de reacción son propietarias y no se han divulgado completamente en la literatura públicamente disponible. se sabe que la síntesis involucra el uso de varios reactivos orgánicos y catalizadores para lograr la estructura química deseada .
Métodos de Producción Industrial
La producción industrial de RGX-202 probablemente involucraría la síntesis a gran escala utilizando condiciones de reacción optimizadas para garantizar un alto rendimiento y pureza. Este proceso incluiría medidas rigurosas de control de calidad para cumplir con los estándares regulatorios para compuestos farmacéuticos. Los métodos de producción también implicarían el uso de técnicas avanzadas de purificación como la cromatografía y la cristalización .
Análisis De Reacciones Químicas
Tipos de Reacciones
RGX-202 experimenta varios tipos de reacciones químicas, incluyendo:
Oxidación: RGX-202 puede oxidarse bajo condiciones específicas para formar varios derivados oxidados.
Reducción: El compuesto también puede experimentar reacciones de reducción, lo que lleva a la formación de productos reducidos.
Reactivos y Condiciones Comunes
Oxidación: Se pueden utilizar agentes oxidantes comunes como el peróxido de hidrógeno o el permanganato de potasio.
Reducción: Los agentes reductores como el borohidruro de sodio o el hidruro de aluminio y litio se emplean típicamente.
Sustitución: Se pueden utilizar varios nucleófilos o electrófilos dependiendo de la reacción de sustitución deseada.
Productos Principales Formados
Los productos principales formados a partir de estas reacciones dependen de los reactivos y condiciones específicos utilizados. Por ejemplo, la oxidación puede producir derivados oxidados con grupos funcionales alterados, mientras que la reducción puede producir formas reducidas de RGX-202 con diferentes propiedades químicas .
Comparación Con Compuestos Similares
Compuestos Similares
Sarepta ElevidysTM SRP-9001: Otra terapia génica diseñada para entregar un transgén para una microdistrofina con elementos funcionales similares a RGX-202.
Pfizer PF-06939926: Una terapia génica que se dirige a la misma vía que RGX-202.
Solid Biosciences SGT-003: Otra terapia génica de investigación con un mecanismo de acción similar.
Unicidad de RGX-202
RGX-202 es único entre su clase de compuestos debido a su objetivo específico del transportador de creatina SLC6A8. Este enfoque dirigido permite una interrupción más precisa del metabolismo energético celular, lo que lo convierte en un candidato prometedor para tratar enfermedades que dependen de esta vía. Además, RGX-202 ha mostrado un perfil de seguridad favorable en estudios clínicos, con efectos adversos mínimos .
Actividad Biológica
3-Guanidinopropionic acid (GPA) is a creatine analogue that has garnered attention for its diverse biological activities, particularly in the context of metabolic regulation, cardiovascular health, and muscle physiology. This article provides a comprehensive overview of the biological activity of GPA, including its mechanisms of action, effects on metabolic disorders, and implications for therapeutic applications.
3-Guanidinopropionic acid is characterized by its molecular formula and a molecular weight of 131.1 g/mol. It is synthesized through the transamidination of L-arginine and β-alanine. GPA acts primarily as a competitive inhibitor of the creatine transporter, which leads to a decrease in intracellular creatine and phosphocreatine levels across various tissues, including skeletal muscle, heart, and brain .
- Creatine Transport Inhibition : GPA competes with creatine for uptake through the creatine transporter, leading to reduced levels of creatine and phosphocreatine in muscle tissues .
- Metabolic Shift : In skeletal muscle, GPA induces a shift from glycolytic to oxidative metabolism, enhancing glucose uptake and increasing fatigue tolerance .
- Cardiovascular Effects : Studies indicate that GPA may lower blood pressure by modulating creatine kinase activity, thus affecting ATP regeneration in cardiac tissues .
1. Insulin Sensitivity and Weight Loss
GPA has been shown to improve insulin sensitivity and promote selective weight loss from adipose tissue in animal models of non-insulin-dependent diabetes mellitus (NIDDM). This effect is attributed to its ability to enhance glucose uptake in muscle tissues while reducing plasma insulin levels .
2. Effects on Muscle Physiology
In animal studies, chronic administration of GPA resulted in significant reductions in total creatine (up to 86.7%), phosphocreatine (up to 79.7%), and ATP levels (up to 38.8%) in skeletal muscle . This depletion leads to:
- Increased reliance on oxidative metabolism.
- Enhanced endurance during physical activities.
- Modest reductions in myocardial contractility without affecting cardiac output .
Case Studies and Clinical Trials
Several studies have explored the safety and efficacy of GPA in human subjects:
- First-in-Human Trial : A study involving 24 healthy men assessed the tolerability of low-dose GPA (100 mg daily) over one week. Results indicated that GPA was well tolerated with no serious adverse events reported. Plasma levels of GPA increased significantly compared to placebo .
- Animal Models : In obese diabetic ob/ob mice, GPA administration resulted in significant weight loss primarily from adipose tissue, confirming its potential as an anti-obesity agent .
Summary of Key Findings
Future Directions
The promising biological activities of 3-Guanidinopropionic acid suggest several avenues for future research:
- Pharmacokinetics and Pharmacodynamics : Further studies are needed to explore the pharmacokinetic profiles at varying doses in humans.
- Therapeutic Applications : Investigating GPA as a potential treatment for metabolic disorders such as obesity and diabetes.
- Long-term Effects : Understanding the long-term implications of GPA supplementation on muscle health and cardiovascular function.
Propiedades
IUPAC Name |
3-(diaminomethylideneamino)propanoic acid | |
---|---|---|
Source | PubChem | |
URL | https://pubchem.ncbi.nlm.nih.gov | |
Description | Data deposited in or computed by PubChem | |
InChI |
InChI=1S/C4H9N3O2/c5-4(6)7-2-1-3(8)9/h1-2H2,(H,8,9)(H4,5,6,7) | |
Source | PubChem | |
URL | https://pubchem.ncbi.nlm.nih.gov | |
Description | Data deposited in or computed by PubChem | |
InChI Key |
KMXXSJLYVJEBHI-UHFFFAOYSA-N | |
Source | PubChem | |
URL | https://pubchem.ncbi.nlm.nih.gov | |
Description | Data deposited in or computed by PubChem | |
Canonical SMILES |
C(CN=C(N)N)C(=O)O | |
Source | PubChem | |
URL | https://pubchem.ncbi.nlm.nih.gov | |
Description | Data deposited in or computed by PubChem | |
Molecular Formula |
C4H9N3O2 | |
Source | PubChem | |
URL | https://pubchem.ncbi.nlm.nih.gov | |
Description | Data deposited in or computed by PubChem | |
DSSTOX Substance ID |
DTXSID40188795 | |
Record name | Guanidinopropionic acid | |
Source | EPA DSSTox | |
URL | https://comptox.epa.gov/dashboard/DTXSID40188795 | |
Description | DSSTox provides a high quality public chemistry resource for supporting improved predictive toxicology. | |
Molecular Weight |
131.13 g/mol | |
Source | PubChem | |
URL | https://pubchem.ncbi.nlm.nih.gov | |
Description | Data deposited in or computed by PubChem | |
Physical Description |
Solid | |
Record name | Beta-Guanidinopropionic acid | |
Source | Human Metabolome Database (HMDB) | |
URL | http://www.hmdb.ca/metabolites/HMDB0013222 | |
Description | The Human Metabolome Database (HMDB) is a freely available electronic database containing detailed information about small molecule metabolites found in the human body. | |
Explanation | HMDB is offered to the public as a freely available resource. Use and re-distribution of the data, in whole or in part, for commercial purposes requires explicit permission of the authors and explicit acknowledgment of the source material (HMDB) and the original publication (see the HMDB citing page). We ask that users who download significant portions of the database cite the HMDB paper in any resulting publications. | |
CAS No. |
353-09-3 | |
Record name | β-Guanidinopropionic acid | |
Source | CAS Common Chemistry | |
URL | https://commonchemistry.cas.org/detail?cas_rn=353-09-3 | |
Description | CAS Common Chemistry is an open community resource for accessing chemical information. Nearly 500,000 chemical substances from CAS REGISTRY cover areas of community interest, including common and frequently regulated chemicals, and those relevant to high school and undergraduate chemistry classes. This chemical information, curated by our expert scientists, is provided in alignment with our mission as a division of the American Chemical Society. | |
Explanation | The data from CAS Common Chemistry is provided under a CC-BY-NC 4.0 license, unless otherwise stated. | |
Record name | Guanidinopropionic acid | |
Source | ChemIDplus | |
URL | https://pubchem.ncbi.nlm.nih.gov/substance/?source=chemidplus&sourceid=0000353093 | |
Description | ChemIDplus is a free, web search system that provides access to the structure and nomenclature authority files used for the identification of chemical substances cited in National Library of Medicine (NLM) databases, including the TOXNET system. | |
Record name | Guanidinopropionic acid | |
Source | EPA DSSTox | |
URL | https://comptox.epa.gov/dashboard/DTXSID40188795 | |
Description | DSSTox provides a high quality public chemistry resource for supporting improved predictive toxicology. | |
Record name | 3-guanidinopropionic acid | |
Source | European Chemicals Agency (ECHA) | |
URL | https://echa.europa.eu/substance-information/-/substanceinfo/100.005.937 | |
Description | The European Chemicals Agency (ECHA) is an agency of the European Union which is the driving force among regulatory authorities in implementing the EU's groundbreaking chemicals legislation for the benefit of human health and the environment as well as for innovation and competitiveness. | |
Explanation | Use of the information, documents and data from the ECHA website is subject to the terms and conditions of this Legal Notice, and subject to other binding limitations provided for under applicable law, the information, documents and data made available on the ECHA website may be reproduced, distributed and/or used, totally or in part, for non-commercial purposes provided that ECHA is acknowledged as the source: "Source: European Chemicals Agency, http://echa.europa.eu/". Such acknowledgement must be included in each copy of the material. ECHA permits and encourages organisations and individuals to create links to the ECHA website under the following cumulative conditions: Links can only be made to webpages that provide a link to the Legal Notice page. | |
Record name | Ompenaclid | |
Source | FDA Global Substance Registration System (GSRS) | |
URL | https://gsrs.ncats.nih.gov/ginas/app/beta/substances/UL1984YRKA | |
Description | The FDA Global Substance Registration System (GSRS) enables the efficient and accurate exchange of information on what substances are in regulated products. Instead of relying on names, which vary across regulatory domains, countries, and regions, the GSRS knowledge base makes it possible for substances to be defined by standardized, scientific descriptions. | |
Explanation | Unless otherwise noted, the contents of the FDA website (www.fda.gov), both text and graphics, are not copyrighted. They are in the public domain and may be republished, reprinted and otherwise used freely by anyone without the need to obtain permission from FDA. Credit to the U.S. Food and Drug Administration as the source is appreciated but not required. | |
Retrosynthesis Analysis
AI-Powered Synthesis Planning: Our tool employs the Template_relevance Pistachio, Template_relevance Bkms_metabolic, Template_relevance Pistachio_ringbreaker, Template_relevance Reaxys, Template_relevance Reaxys_biocatalysis model, leveraging a vast database of chemical reactions to predict feasible synthetic routes.
One-Step Synthesis Focus: Specifically designed for one-step synthesis, it provides concise and direct routes for your target compounds, streamlining the synthesis process.
Accurate Predictions: Utilizing the extensive PISTACHIO, BKMS_METABOLIC, PISTACHIO_RINGBREAKER, REAXYS, REAXYS_BIOCATALYSIS database, our tool offers high-accuracy predictions, reflecting the latest in chemical research and data.
Strategy Settings
Precursor scoring | Relevance Heuristic |
---|---|
Min. plausibility | 0.01 |
Model | Template_relevance |
Template Set | Pistachio/Bkms_metabolic/Pistachio_ringbreaker/Reaxys/Reaxys_biocatalysis |
Top-N result to add to graph | 6 |
Feasible Synthetic Routes
Descargo de responsabilidad e información sobre productos de investigación in vitro
Tenga en cuenta que todos los artículos e información de productos presentados en BenchChem están destinados únicamente con fines informativos. Los productos disponibles para la compra en BenchChem están diseñados específicamente para estudios in vitro, que se realizan fuera de organismos vivos. Los estudios in vitro, derivados del término latino "in vidrio", involucran experimentos realizados en entornos de laboratorio controlados utilizando células o tejidos. Es importante tener en cuenta que estos productos no se clasifican como medicamentos y no han recibido la aprobación de la FDA para la prevención, tratamiento o cura de ninguna condición médica, dolencia o enfermedad. Debemos enfatizar que cualquier forma de introducción corporal de estos productos en humanos o animales está estrictamente prohibida por ley. Es esencial adherirse a estas pautas para garantizar el cumplimiento de los estándares legales y éticos en la investigación y experimentación.