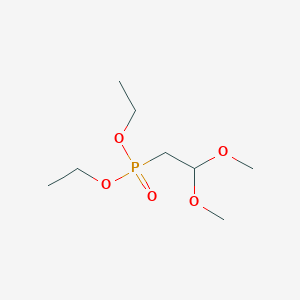
Diethyl (2,2-dimethoxyethyl)phosphonate
Descripción general
Descripción
Diethyl (2,2-dimethoxyethyl)phosphonate (CAS: 7598-61-0) is an organophosphorus compound with the molecular formula C₁₀H₂₃O₅P and a molecular weight of 254.26 g/mol . Structurally, it features a phosphonate core linked to a 2,2-dimethoxyethyl group, which introduces ether functionalities that influence its solubility and reactivity. The compound is also known by synonyms such as Diethyl phosphonoacetaldehyde diethyl acetal and Diethyl-(2,2-diethoxyethyl)phosphonat . Its synthesis typically involves nucleophilic substitution or condensation reactions, often starting with diethyl phosphite and a dimethoxyethyl halide precursor.
Mecanismo De Acción
Target of Action
Diethyl (2,2-dimethoxyethyl)phosphonate is an organophosphorus compound It has been used as a stable proton transfer reagent for kinetic studies of proton-transfer reactions in organic solvents .
Mode of Action
The mode of action of this compound involves its role as a proton transfer reagent . It interacts with its targets by facilitating proton-transfer reactions, which are fundamental to many chemical and biological processes.
Pharmacokinetics
It is known that the compound is a colorless liquid that is soluble in organic solvents , which may influence its absorption and distribution.
Action Environment
The action, efficacy, and stability of this compound can be influenced by various environmental factors. For instance, its solubility in organic solvents suggests that the presence and nature of such solvents in the environment could impact its action and efficacy.
Actividad Biológica
Diethyl (2,2-dimethoxyethyl)phosphonate is a phosphonate compound that has garnered attention for its diverse biological activities. This article explores its synthesis, biological effects, and potential applications, drawing from recent studies and findings.
Chemical Structure and Synthesis
This compound is characterized by the presence of two methoxy groups attached to a 2-ethyl phosphonate backbone. The synthesis typically involves the reaction of diethyl phosphite with 2,2-dimethoxyethyl bromide or similar alkylating agents under basic conditions. The general reaction scheme can be represented as follows:
Antimicrobial Properties
Recent studies have highlighted the antimicrobial potential of this compound. In vitro assays demonstrated significant activity against various bacterial strains, including Escherichia coli and Staphylococcus aureus. The minimal inhibitory concentration (MIC) values indicate that this compound exhibits a higher selectivity and efficacy compared to traditional antibiotics like ciprofloxacin and cloxacillin .
Table 1: Antimicrobial Activity of this compound
Bacterial Strain | MIC (µg/mL) | Comparison Antibiotic | MIC (µg/mL) |
---|---|---|---|
E. coli K12 | 32 | Ciprofloxacin | 64 |
S. aureus | 16 | Cloxacillin | 32 |
Cytotoxicity Studies
Cytotoxicity assays using human cell lines have shown that this compound exhibits moderate cytotoxic effects. The compound was tested against several cancer cell lines, including human promyelocytic leukemia (HL-60) and mouse fibroblast (NIH/3T3) cells. The IC50 values indicated that while some derivatives showed promising activity, further structural modifications may enhance their efficacy .
Table 2: Cytotoxicity of this compound on Cancer Cell Lines
Cell Line | IC50 (µM) |
---|---|
HL-60 | 25 |
NIH/3T3 | 30 |
The biological activity of this compound is believed to be linked to its ability to interact with cellular membranes and induce oxidative stress within bacterial cells. This interaction can lead to membrane disruption and subsequent cell death. Additionally, the presence of the phosphonate group may enhance metabolic stability and bioavailability in biological systems .
Case Studies
- Antibacterial Efficacy : A study conducted on various phosphonates including this compound revealed that it significantly reduced the viability of E. coli strains through mechanisms involving DNA damage and oxidative stress induction .
- Anticancer Potential : In another investigation focusing on its anticancer properties, this compound demonstrated selective cytotoxicity towards HL-60 cells with minimal effects on normal fibroblast cells. This selectivity suggests potential for development as an anticancer agent .
Aplicaciones Científicas De Investigación
DEPN exhibits significant flame retardant properties, making it an effective additive for various materials. Studies have demonstrated its ability to reduce flammability and smoke generation in cotton fabrics .
Case Study: Flame Retardancy in Textiles
- Study : Wang et al. (2012) investigated the efficacy of DEPN as a flame retardant for cotton fabrics.
- Findings : The treated fabrics showed a marked decrease in flammability compared to untreated samples, with reduced smoke production during combustion.
Applications in Polymer Chemistry
DEPN is utilized as a plasticizer in polymer formulations, enhancing flexibility and processability. Its incorporation into poly(ether-imide)s has been studied, resulting in improved mechanical properties .
Table 2: Properties of DEPN-Modified Polymers
Polymer Type | Modification Type | Effect on Properties |
---|---|---|
Poly(ether-imide) | Incorporation of DEPN | Increased flexibility |
Synthetic Reagent Applications
DEPN is also employed as a synthetic reagent in various chemical reactions, including:
- Asymmetric Michael addition
- Gem-chlorofluorination
- Cyclocondensation reactions
- Horner-Wadsworth-Emmons reactions .
Table 3: Synthetic Reactions Involving DEPN
Reaction Type | Application |
---|---|
Asymmetric Michael Addition | Synthesis of β-oxo phosphonates |
Gem-Chlorofluorination | Functionalization of products |
Cyclocondensation | Production of arylphosphonates |
Q & A
Basic Research Questions
Q. What are the standard laboratory synthesis protocols for Diethyl (2,2-dimethoxyethyl)phosphonate?
- Methodology : The compound is synthesized via diazotransfer reactions using sulfonyl azides (e.g., p-nitrobenzenesulfonyl azide) under anhydrous conditions. A typical procedure involves reacting a precursor aldehyde with diethyl phosphite in tetrahydrofuran (THF) at 0°C, followed by diazotransfer to introduce the diazo group. The sulfonamide byproduct is removed via filtration, and the product is purified by column chromatography (silica gel, ethyl acetate/petroleum ether) .
- Key Data : Yields range from 65–85% depending on solvent choice and stoichiometry. THF is preferred for optimal solubility and reaction homogeneity.
Q. Which spectroscopic techniques are critical for characterizing this compound?
- 31P NMR : The phosphorus environment is analyzed using 31P NMR, with chemical shifts typically observed between δ +15 to +25 ppm. Coupling patterns (e.g., JPH ≈ 20–25 Hz) confirm the phosphonate structure .
- X-ray Diffraction : Single-crystal X-ray analysis resolves bond angles and torsional distortions (e.g., nitro groups deviating ~30° from aromatic planes) to validate molecular geometry .
Q. What safety protocols are essential for handling this compound?
- Risk Mitigation : Conduct hazard assessments per Prudent Practices in the Laboratory (National Academies Press, 2011). Use explosion-proof equipment, maintain inert atmospheres (N2/Ar), and employ personal protective equipment (PPE) due to the compound’s diazo group instability .
Advanced Research Questions
Q. How can reaction conditions be optimized to improve yield and purity?
- Solvent Optimization : THF enhances reagent solubility, but dichloromethane (DCM) may reduce side reactions in temperature-sensitive steps.
- Stoichiometric Adjustments : A 1.2:1 molar ratio of sulfonyl azide to aldehyde precursor minimizes unreacted intermediates. Excess azide increases diazo group incorporation but risks byproduct formation .
- Data Contradictions : Lower yields (<50%) in DCM vs. THF (75–85%) highlight solvent polarity’s role in stabilizing transition states.
Q. How do analytical challenges arise in characterizing reaction intermediates?
- Diazo Stability : Diazocarbonyl intermediates are light- and heat-sensitive. Use in-situ monitoring (e.g., FTIR or low-temperature NMR) to track transient species.
- Resolution of NMR Overlaps : Apply 2D NMR (HSQC, HMBC) to distinguish overlapping signals in complex mixtures, particularly for phosphonate and diazo protons .
Q. What strategies resolve discrepancies in reported NMR chemical shifts?
- Decoupling Techniques : 1H-decoupled 31P NMR simplifies spectra by eliminating splitting from adjacent protons.
- Reference Standards : Compare shifts with structurally analogous compounds (e.g., diethyl phosphonate: δ +22 ppm) to validate assignments .
Q. How do synthetic route variations impact product stability and scalability?
- Alternative Routes : Microwave-assisted synthesis reduces reaction times but may compromise diazo group integrity.
- Scalability : Batch-wise diazotransfer in THF at 0°C ensures reproducible yields (>80%) at 10–50 mmol scales. Larger scales require rigorous temperature control to prevent exothermic decomposition .
Comparación Con Compuestos Similares
Comparison with Structural Analogs
Diethyl 2-Oxo-2-Arylethylphosphonates (e.g., Compounds 13–18)
- Structure : These compounds contain aromatic rings (e.g., 3,5-dimethoxyphenyl or 3,4,5-trimethoxyphenyl) linked to a ketone-phosphonate group .
- Reactivity : The electron-withdrawing aryl groups enhance electrophilicity at the α-carbon, making these compounds reactive toward nucleophiles. For example, they participate in Michael additions or act as intermediates in heterocycle synthesis.
- Applications : Used in medicinal chemistry for antimalarial or antitumor agent synthesis due to their aromatic pharmacophores .
Diethyl (2-Chloroethyl)phosphonate
- Structure : Features a chloroethyl group (C₆H₁₄ClO₃P) .
- Reactivity : The chlorine atom facilitates nucleophilic substitution (e.g., SN2 reactions), enabling cross-coupling or alkylation processes.
- Contrast : Unlike the dimethoxyethyl group, the chloro substituent increases polarity and reduces stability under basic conditions .
Diethyl (Dichloromethyl)phosphonate
- Structure : Contains a dichloromethyl group (C₃H₇Cl₂O₃P) .
- Reactivity : The dichloromethyl group acts as a precursor for ylide generation, useful in olefination reactions (e.g., synthesizing 4-methoxystyrene) .
- Applications : Primarily employed in organic synthesis for C–C bond formation.
Electronic and Steric Influences
- Diethyl (2-Oxobutyl)phosphonate (C₈H₁₇O₄P): The ketone group at the α-position increases electrophilicity, enabling condensation reactions (e.g., Knoevenagel) .
- Methyl Diethylphosphonoacetate (C₇H₁₅O₅P): The carbomethoxy group (-COOMe) enhances electron-withdrawing effects, stabilizing enolate intermediates for use in Horner-Wadsworth-Emmons reactions .
- Diethyl (2,2-Dimethoxyethyl)phosphonate : The dimethoxyethyl group provides steric bulk and electron-donating effects, improving solubility in polar aprotic solvents (e.g., DMF or acetonitrile) .
Data Tables
Table 1: Structural and Physical Properties
Métodos De Preparación
Michaelis-Arbuzov Reaction: Alkylation of Triethyl Phosphite
The Michaelis-Arbuzov reaction remains a cornerstone in phosphonate synthesis, leveraging trialkyl phosphites and alkyl halides. For diethyl (2,2-dimethoxyethyl)phosphonate, this involves reacting triethyl phosphite with 2,2-dimethoxyethyl bromide under thermal conditions . The mechanism proceeds via nucleophilic attack of the phosphite’s oxygen on the electrophilic carbon of the alkyl halide, displacing bromide and forming the phosphonate ester.
Reaction Conditions :
-
Temperature : 80–120°C (optimized to prevent decomposition of the dimethoxyethyl group) .
-
Solvent : Solvent-free or inert aprotic media (e.g., toluene) to enhance electrophilicity.
-
Catalyst : None required, though Lewis acids like FeCl₃ may accelerate the reaction .
Yield Considerations :
-
Theoretical yields exceed 90% when using high-purity halides and anhydrous conditions.
-
Practical yields often range between 75–85% due to competing elimination or hydrolysis .
Limitations :
-
Requires stoichiometric alkyl halides, which may be synthetically laborious to prepare.
-
Geminal dimethoxy groups introduce steric hindrance, slowing reaction kinetics .
Nucleophilic Substitution with Diethyl Phosphite Salts
An alternative route employs the sodium salt of diethyl phosphite (Na⁺[P(O)(OEt)₂]⁻) reacting with 2,2-dimethoxyethyl bromide. This method circumvents the need for triethyl phosphite, instead utilizing the phosphite’s conjugate base for enhanced nucleophilicity .
Key Steps :
-
Generation of Phosphite Anion :
-
Alkylation :
-
The anion attacks the alkyl halide at 0–25°C, minimizing side reactions.
-
Optimization Parameters :
-
Base Selection : KF/Al₂O₃ mixtures improve solubility and reaction homogeneity .
-
Solvent : Tetrahydrofuran (THF) or dimethyl sulfoxide (DMSO) enhances ion pair separation .
-
Atmosphere : Inert gas (N₂ or Ar) prevents oxidation of the phosphite anion.
Performance Metrics :
-
Yields: 70–90%, contingent on halide reactivity and base strength .
-
Purity: Post-reaction distillation or column chromatography isolates the product .
Metal-Catalyzed Coupling Reactions
Transition metal catalysis offers a versatile pathway, particularly for substrates resistant to conventional alkylation. Copper(I) triflate (CuOTf) and iron(III) chloride (FeCl₃) synergistically mediate couplings between diethyl phosphite and dimethoxyethyl precursors .
Mechanistic Insights :
-
Oxidative Addition : Cu(I) activates the C–X bond in halides or pseudohalides.
-
Transmetalation : Fe(III) facilitates phosphite coordination to the metal center.
-
Reductive Elimination : Forms the C–P bond, releasing the phosphonate .
Protocol :
Efficiency :
Hydrophosphonylation of Dimethoxy-Substituted Alkenes
Radical-mediated hydrophosphonylation adds diethyl phosphite across electron-deficient alkenes, such as 2,2-dimethoxyethyl acrylate. This method exploits the phosphite’s ability to undergo radical chain propagation .
Reaction Design :
-
Initiator : Di-tert-butyl peroxide (DTBP) generates phosphite radicals at 80–100°C .
-
Regioselectivity : Anti-Markovnikov addition dominates due to radical stability .
Challenges :
-
Dimethoxy groups may sterically hinder alkene accessibility.
-
Competing polymerization requires careful initiator dosing .
Two-Step Synthesis via Hydroxyphosphonate Intermediates
A less direct route involves forming a hydroxyphosphonate intermediate through Abramov addition to a ketone, followed by deoxygenation. For example, reacting diethyl phosphite with dimethoxyacetone yields a secondary alcohol, which is reduced using LiAlH₄ .
Steps :
-
Abramov Addition :
-
Deoxygenation :
Drawbacks :
-
Multi-step synthesis lowers overall yield (30–56%).
Comparative Analysis of Synthetic Methods
Method | Yield (%) | Reaction Time | Complexity | Scalability |
---|---|---|---|---|
Michaelis-Arbuzov | 75–85 | 6–12 h | Low | High |
Nucleophilic Substitution | 70–90 | 2–6 h | Moderate | Moderate |
Metal-Catalyzed Coupling | 60–75 | 24 h | High | Low |
Hydrophosphonylation | 50–65 | 8–16 h | Moderate | Moderate |
Two-Step Synthesis | 30–56 | 48 h | High | Low |
Key Observations :
-
The Michaelis-Arbuzov and nucleophilic substitution routes offer the best balance of yield and practicality .
-
Metal-catalyzed methods, while innovative, suffer from extended reaction times and lower scalability .
Experimental Considerations and Optimization
Critical Parameters :
-
Moisture Control : All reactions require anhydrous conditions to prevent hydrolysis of intermediates .
-
Temperature Gradients : Gradual heating (e.g., 2°C/min) minimizes decomposition of heat-sensitive dimethoxy groups .
-
Purification : Distillation (bp 120–122°C) or silica gel chromatography (petroleum ether/ethyl acetate) ensures product purity .
Troubleshooting :
Propiedades
IUPAC Name |
2-diethoxyphosphoryl-1,1-dimethoxyethane | |
---|---|---|
Source | PubChem | |
URL | https://pubchem.ncbi.nlm.nih.gov | |
Description | Data deposited in or computed by PubChem | |
InChI |
InChI=1S/C8H19O5P/c1-5-12-14(9,13-6-2)7-8(10-3)11-4/h8H,5-7H2,1-4H3 | |
Source | PubChem | |
URL | https://pubchem.ncbi.nlm.nih.gov | |
Description | Data deposited in or computed by PubChem | |
InChI Key |
XMORGWRBGODJMM-UHFFFAOYSA-N | |
Source | PubChem | |
URL | https://pubchem.ncbi.nlm.nih.gov | |
Description | Data deposited in or computed by PubChem | |
Canonical SMILES |
CCOP(=O)(CC(OC)OC)OCC | |
Source | PubChem | |
URL | https://pubchem.ncbi.nlm.nih.gov | |
Description | Data deposited in or computed by PubChem | |
Molecular Formula |
C8H19O5P | |
Source | PubChem | |
URL | https://pubchem.ncbi.nlm.nih.gov | |
Description | Data deposited in or computed by PubChem | |
DSSTOX Substance ID |
DTXSID50390625 | |
Record name | Diethyl (2,2-dimethoxyethyl)phosphonate | |
Source | EPA DSSTox | |
URL | https://comptox.epa.gov/dashboard/DTXSID50390625 | |
Description | DSSTox provides a high quality public chemistry resource for supporting improved predictive toxicology. | |
Molecular Weight |
226.21 g/mol | |
Source | PubChem | |
URL | https://pubchem.ncbi.nlm.nih.gov | |
Description | Data deposited in or computed by PubChem | |
CAS No. |
17053-13-3 | |
Record name | Diethyl (2,2-dimethoxyethyl)phosphonate | |
Source | EPA DSSTox | |
URL | https://comptox.epa.gov/dashboard/DTXSID50390625 | |
Description | DSSTox provides a high quality public chemistry resource for supporting improved predictive toxicology. | |
Descargo de responsabilidad e información sobre productos de investigación in vitro
Tenga en cuenta que todos los artículos e información de productos presentados en BenchChem están destinados únicamente con fines informativos. Los productos disponibles para la compra en BenchChem están diseñados específicamente para estudios in vitro, que se realizan fuera de organismos vivos. Los estudios in vitro, derivados del término latino "in vidrio", involucran experimentos realizados en entornos de laboratorio controlados utilizando células o tejidos. Es importante tener en cuenta que estos productos no se clasifican como medicamentos y no han recibido la aprobación de la FDA para la prevención, tratamiento o cura de ninguna condición médica, dolencia o enfermedad. Debemos enfatizar que cualquier forma de introducción corporal de estos productos en humanos o animales está estrictamente prohibida por ley. Es esencial adherirse a estas pautas para garantizar el cumplimiento de los estándares legales y éticos en la investigación y experimentación.