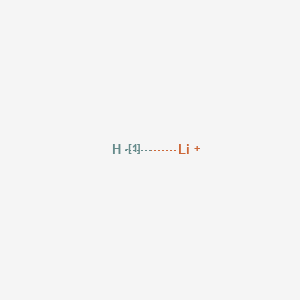
Deuteruro de litio
Descripción general
Descripción
Lithium deuteride (LiD) is a compound composed of lithium and deuterium atoms, which is widely used in scientific research and laboratory experiments. It is a white solid with a melting point of 533°C and a boiling point of 1,400°C. LiD is a versatile compound with a wide range of applications in nuclear, atomic, and chemical research.
Aplicaciones Científicas De Investigación
Desarrollo de la Bomba de Hidrógeno
En el ámbito militar, el deuteruro de litio es famoso por su uso como combustible de fusión sólido para las bombas de hidrógeno . La reacción de un neutrón con litio es un método conveniente para producir tritio, que es esencial para la reacción termonuclear en las bombas de hidrógeno. El uso de this compound simplifica el proceso al evitar sistemas de congelación complejos.
Marcado Isotópico y Reacciones de Intercambio
En la investigación química, el this compound se utiliza para el marcado isotópico y las reacciones de intercambio de deuterio . Estas técnicas son vitales para comprender las vías y los mecanismos químicos, particularmente en moléculas orgánicas complejas y productos farmacéuticos.
Estudios de Efectos Isotópicos del Deuterio
El estudio de los efectos isotópicos del deuterio es otra aplicación importante del this compound . Estos efectos se observan en varios procesos químicos, biológicos y físicos, proporcionando información sobre la cinética de reacción y la dinámica molecular.
Síntesis de Compuestos Organometálicos
El this compound sirve como fuente de deuterio para la síntesis de varios compuestos organometálicos . Estos compuestos son fundamentales en la investigación y el desarrollo de nuevos materiales con posibles aplicaciones en electrónica, catálisis y más.
Mecanismo De Acción
Target of Action
Lithium deuteride primarily targets nuclear reactions, specifically in the context of nuclear fusion . It is used as a fusion fuel in thermonuclear weapons . The compound’s primary role is to facilitate the fusion of deuterium and tritium, two isotopes of hydrogen .
Mode of Action
The mode of action of lithium deuteride involves a series of nuclear reactions. Initially, the fission reaction of the primary releases gamma and x-ray, neutrons, and heat to produce the high temperatures required for the following fusion reactions . The fuel for the fusion is lithium deuteride. The neutrons react with lithium to produce tritium, and alpha radiation .
Biochemical Pathways
Instead, it is involved in nuclear reactions, specifically the fusion of deuterium and tritium . This fusion reaction results in the release of a large amount of energy, which is the primary “downstream effect” of this process .
Pharmacokinetics
Instead, it undergoes nuclear reactions to produce energy .
Result of Action
The result of lithium deuteride’s action is the production of a large amount of energy through nuclear fusion . This energy is released in the form of gamma rays, kinetic energy, and other forms of radiation . In the context of its use in thermonuclear weapons, this energy release results in a large explosion .
Action Environment
The action of lithium deuteride is influenced by environmental factors such as temperature and pressure . High temperatures and pressures are required to initiate the nuclear fusion reactions in which lithium deuteride participates . Additionally, lithium deuteride is a solid under ambient conditions without cooling and compression, which has low production cost, small size, lightweight, and convenience to carry .
Safety and Hazards
Direcciones Futuras
Jefferson Lab is on the ground level of a multi-year, combined research venture to measure spin-polarized fusion (SPF) . If achieved, SPF will lower the requirements for the ignition of a burning plasma in a fusion reactor . Atomic nuclei have a behavior akin to a spinning top. Low temperatures and high magnetic fields can be used to “polarize” all the nuclei in a fuel pellet by forcing them to all spin in the same direction .
Propiedades
IUPAC Name |
lithium;deuteride | |
---|---|---|
Source | PubChem | |
URL | https://pubchem.ncbi.nlm.nih.gov | |
Description | Data deposited in or computed by PubChem | |
InChI |
InChI=1S/Li.H/q+1;-1/i;1+1 | |
Source | PubChem | |
URL | https://pubchem.ncbi.nlm.nih.gov | |
Description | Data deposited in or computed by PubChem | |
InChI Key |
SRTHRWZAMDZJOS-IEOVAKBOSA-N | |
Source | PubChem | |
URL | https://pubchem.ncbi.nlm.nih.gov | |
Description | Data deposited in or computed by PubChem | |
Canonical SMILES |
[H-].[Li+] | |
Source | PubChem | |
URL | https://pubchem.ncbi.nlm.nih.gov | |
Description | Data deposited in or computed by PubChem | |
Isomeric SMILES |
[2H-].[Li+] | |
Source | PubChem | |
URL | https://pubchem.ncbi.nlm.nih.gov | |
Description | Data deposited in or computed by PubChem | |
Molecular Formula |
HLi | |
Source | PubChem | |
URL | https://pubchem.ncbi.nlm.nih.gov | |
Description | Data deposited in or computed by PubChem | |
DSSTOX Substance ID |
DTXSID501043436 | |
Record name | Lithium deuteride | |
Source | EPA DSSTox | |
URL | https://comptox.epa.gov/dashboard/DTXSID501043436 | |
Description | DSSTox provides a high quality public chemistry resource for supporting improved predictive toxicology. | |
Molecular Weight |
9.0 g/mol | |
Source | PubChem | |
URL | https://pubchem.ncbi.nlm.nih.gov | |
Description | Data deposited in or computed by PubChem | |
Physical Description |
Gray crystals with melting point of 680 deg C; [Hawley] | |
Record name | Lithium deuteride | |
Source | Haz-Map, Information on Hazardous Chemicals and Occupational Diseases | |
URL | https://haz-map.com/Agents/2017 | |
Description | Haz-Map® is an occupational health database designed for health and safety professionals and for consumers seeking information about the adverse effects of workplace exposures to chemical and biological agents. | |
Explanation | Copyright (c) 2022 Haz-Map(R). All rights reserved. Unless otherwise indicated, all materials from Haz-Map are copyrighted by Haz-Map(R). No part of these materials, either text or image may be used for any purpose other than for personal use. Therefore, reproduction, modification, storage in a retrieval system or retransmission, in any form or by any means, electronic, mechanical or otherwise, for reasons other than personal use, is strictly prohibited without prior written permission. | |
CAS RN |
13587-16-1 | |
Record name | Lithium (2H)hydride | |
Source | ChemIDplus | |
URL | https://pubchem.ncbi.nlm.nih.gov/substance/?source=chemidplus&sourceid=0013587161 | |
Description | ChemIDplus is a free, web search system that provides access to the structure and nomenclature authority files used for the identification of chemical substances cited in National Library of Medicine (NLM) databases, including the TOXNET system. | |
Record name | Lithium deuteride | |
Source | EPA DSSTox | |
URL | https://comptox.epa.gov/dashboard/DTXSID501043436 | |
Description | DSSTox provides a high quality public chemistry resource for supporting improved predictive toxicology. | |
Record name | Lithium (2H)hydride | |
Source | European Chemicals Agency (ECHA) | |
URL | https://echa.europa.eu/substance-information/-/substanceinfo/100.033.638 | |
Description | The European Chemicals Agency (ECHA) is an agency of the European Union which is the driving force among regulatory authorities in implementing the EU's groundbreaking chemicals legislation for the benefit of human health and the environment as well as for innovation and competitiveness. | |
Explanation | Use of the information, documents and data from the ECHA website is subject to the terms and conditions of this Legal Notice, and subject to other binding limitations provided for under applicable law, the information, documents and data made available on the ECHA website may be reproduced, distributed and/or used, totally or in part, for non-commercial purposes provided that ECHA is acknowledged as the source: "Source: European Chemicals Agency, http://echa.europa.eu/". Such acknowledgement must be included in each copy of the material. ECHA permits and encourages organisations and individuals to create links to the ECHA website under the following cumulative conditions: Links can only be made to webpages that provide a link to the Legal Notice page. | |
Descargo de responsabilidad e información sobre productos de investigación in vitro
Tenga en cuenta que todos los artículos e información de productos presentados en BenchChem están destinados únicamente con fines informativos. Los productos disponibles para la compra en BenchChem están diseñados específicamente para estudios in vitro, que se realizan fuera de organismos vivos. Los estudios in vitro, derivados del término latino "in vidrio", involucran experimentos realizados en entornos de laboratorio controlados utilizando células o tejidos. Es importante tener en cuenta que estos productos no se clasifican como medicamentos y no han recibido la aprobación de la FDA para la prevención, tratamiento o cura de ninguna condición médica, dolencia o enfermedad. Debemos enfatizar que cualquier forma de introducción corporal de estos productos en humanos o animales está estrictamente prohibida por ley. Es esencial adherirse a estas pautas para garantizar el cumplimiento de los estándares legales y éticos en la investigación y experimentación.