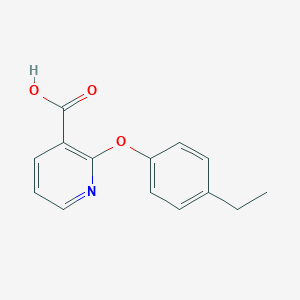
Ácido 2-(4-etilfenoxi)nicotínico
- Haga clic en CONSULTA RÁPIDA para recibir una cotización de nuestro equipo de expertos.
- Con productos de calidad a un precio COMPETITIVO, puede centrarse más en su investigación.
Descripción general
Descripción
2-(4-Ethylphenoxy)nicotinic acid is an organic compound with the molecular formula C14H13NO3Nicotinic acid and its derivatives are known for their wide range of biological activities and applications in various fields, including medicine, chemistry, and industry .
Aplicaciones Científicas De Investigación
2-(4-Ethylphenoxy)nicotinic acid has diverse applications in scientific research:
Chemistry: Used as a building block for the synthesis of more complex organic molecules.
Biology: Investigated for its potential as an antibacterial and antifungal agent.
Medicine: Explored for its anti-inflammatory and analgesic properties.
Industry: Utilized in the development of new materials and as a precursor in the synthesis of pharmaceuticals .
Mecanismo De Acción
Target of Action
The primary target of 2-(4-Ethylphenoxy)nicotinic acid, a derivative of nicotinic acid, is the nicotinic acetylcholine receptors (nAChRs) . These receptors are found in the brain and play a crucial role in neuronal signaling .
Mode of Action
2-(4-Ethylphenoxy)nicotinic acid interacts with its targets, the nAChRs, by binding to these receptors . This interaction leads to changes in the properties of the receptors, including their sensitivity to nicotine, permeability to calcium, and propensity to desensitize .
Biochemical Pathways
The compound affects the biochemical pathways related to the metabolism of nicotine . A pyridine ring of nicotine and related alkaloids is derived from nicotinic acid, a primary metabolite in the pathway that supplies nicotinamide adenine dinucleotide (NAD), an important co-factor for oxidation–reduction reactions . In the hybrid pathway, nicotine is catabolized into fumaric acid via 10 steps, which then enters the TCA cycle .
Pharmacokinetics
The pharmacokinetics of nicotinic acid, from which the compound is derived, has been studied . Nicotine equilibrates in the endoplasmic reticulum within 10 seconds of extracellular application and leaves as rapidly after removal from the extracellular solution . The concentration of nicotine in the endoplasmic reticulum is within twofold of the extracellular value .
Result of Action
It is known that 2-substituted aryl derived from nicotinic acid, such as 2–bromo aryl substituents, have shown anti-inflammatory and analgesic efficacy .
Action Environment
The action, efficacy, and stability of 2-(4-Ethylphenoxy)nicotinic acid can be influenced by environmental factors. For instance, the solubility of nicotinic acid in various organic solvents has been analyzed through two linear Gibbs energy relationships to extract compound properties that encode various solute-solvent interactions . This suggests that the action of 2-(4-Ethylphenoxy)nicotinic acid could also be influenced by the solvent environment.
Análisis Bioquímico
Biochemical Properties
2-(4-Ethylphenoxy)nicotinic acid, like its structural relative nicotinic acid, may interact with various enzymes, proteins, and other biomolecules. Specific interactions of 2-(4-Ethylphenoxy)nicotinic acid have not been extensively studied .
Cellular Effects
It’s structurally related compound, nicotinic acid, is known to have significant effects on cell function, including impacts on cell signaling pathways, gene expression, and cellular metabolism .
Molecular Mechanism
Given its structural similarity to nicotinic acid, it may exert its effects at the molecular level through similar mechanisms, such as binding interactions with biomolecules, enzyme inhibition or activation, and changes in gene expression .
Dosage Effects in Animal Models
The effects of varying dosages of 2-(4-Ethylphenoxy)nicotinic acid in animal models have not been extensively studied. Related compounds such as nicotinic acid have been shown to have dosage-dependent effects .
Metabolic Pathways
2-(4-Ethylphenoxy)nicotinic acid may be involved in metabolic pathways similar to those of nicotinic acid, given their structural similarity. Nicotinic acid is a key component in the biosynthesis of NAD, an essential cofactor for many oxidation–reduction reactions .
Transport and Distribution
It’s structurally related compound, nicotinic acid, is known to be transported into cells via specific transporters .
Métodos De Preparación
Synthetic Routes and Reaction Conditions
The synthesis of 2-(4-Ethylphenoxy)nicotinic acid typically involves the reaction of 4-ethylphenol with nicotinic acid under specific conditions. One common method involves the use of a base, such as sodium hydroxide, to deprotonate the phenol, followed by the addition of nicotinic acid. The reaction is usually carried out in an organic solvent like dimethylformamide (DMF) at elevated temperatures .
Industrial Production Methods
On an industrial scale, nicotinic acid derivatives are often produced through the oxidation of 5-ethyl-2-methylpyridine with nitric acid. This method is efficient but generates nitrous oxide as a by-product, which poses environmental challenges. Therefore, green chemistry approaches are being explored to make the process more sustainable .
Análisis De Reacciones Químicas
Types of Reactions
2-(4-Ethylphenoxy)nicotinic acid can undergo various chemical reactions, including:
Oxidation: This reaction typically involves the use of oxidizing agents like potassium permanganate or hydrogen peroxide.
Reduction: Common reducing agents include lithium aluminum hydride (LiAlH4) and sodium borohydride (NaBH4).
Common Reagents and Conditions
Oxidation: Potassium permanganate in an acidic medium.
Reduction: Lithium aluminum hydride in anhydrous ether.
Substitution: Sodium hydroxide in aqueous or alcoholic solutions.
Major Products Formed
The major products formed from these reactions depend on the specific conditions and reagents used. For example, oxidation can yield carboxylic acids, while reduction can produce alcohols or amines .
Comparación Con Compuestos Similares
Similar Compounds
Nicotinic Acid: The parent compound, known for its role in lipid metabolism and cardiovascular health.
Nicotinamide: An amide derivative of nicotinic acid with similar biological activities.
2-Chloronicotinic Acid: Another derivative with distinct chemical properties and applications
Uniqueness
2-(4-Ethylphenoxy)nicotinic acid is unique due to the presence of the ethylphenoxy group, which imparts specific chemical and biological properties. This structural feature differentiates it from other nicotinic acid derivatives and contributes to its distinct reactivity and applications .
Propiedades
IUPAC Name |
2-(4-ethylphenoxy)pyridine-3-carboxylic acid |
Source
|
---|---|---|
Source | PubChem | |
URL | https://pubchem.ncbi.nlm.nih.gov | |
Description | Data deposited in or computed by PubChem | |
InChI |
InChI=1S/C14H13NO3/c1-2-10-5-7-11(8-6-10)18-13-12(14(16)17)4-3-9-15-13/h3-9H,2H2,1H3,(H,16,17) |
Source
|
Source | PubChem | |
URL | https://pubchem.ncbi.nlm.nih.gov | |
Description | Data deposited in or computed by PubChem | |
InChI Key |
RICLKTXAEHRTNZ-UHFFFAOYSA-N |
Source
|
Source | PubChem | |
URL | https://pubchem.ncbi.nlm.nih.gov | |
Description | Data deposited in or computed by PubChem | |
Canonical SMILES |
CCC1=CC=C(C=C1)OC2=C(C=CC=N2)C(=O)O |
Source
|
Source | PubChem | |
URL | https://pubchem.ncbi.nlm.nih.gov | |
Description | Data deposited in or computed by PubChem | |
Molecular Formula |
C14H13NO3 |
Source
|
Source | PubChem | |
URL | https://pubchem.ncbi.nlm.nih.gov | |
Description | Data deposited in or computed by PubChem | |
Molecular Weight |
243.26 g/mol |
Source
|
Source | PubChem | |
URL | https://pubchem.ncbi.nlm.nih.gov | |
Description | Data deposited in or computed by PubChem | |
Descargo de responsabilidad e información sobre productos de investigación in vitro
Tenga en cuenta que todos los artículos e información de productos presentados en BenchChem están destinados únicamente con fines informativos. Los productos disponibles para la compra en BenchChem están diseñados específicamente para estudios in vitro, que se realizan fuera de organismos vivos. Los estudios in vitro, derivados del término latino "in vidrio", involucran experimentos realizados en entornos de laboratorio controlados utilizando células o tejidos. Es importante tener en cuenta que estos productos no se clasifican como medicamentos y no han recibido la aprobación de la FDA para la prevención, tratamiento o cura de ninguna condición médica, dolencia o enfermedad. Debemos enfatizar que cualquier forma de introducción corporal de estos productos en humanos o animales está estrictamente prohibida por ley. Es esencial adherirse a estas pautas para garantizar el cumplimiento de los estándares legales y éticos en la investigación y experimentación.