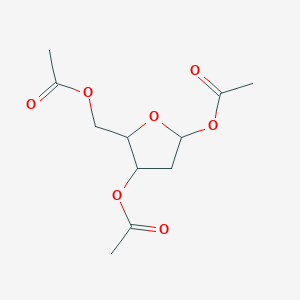
1,3,5-Tri-O-acetyl-2-deoxy-D-erythro-pentofuranose
Descripción general
Descripción
1,3,5-Tri-O-acetyl-2-deoxy-D-erythro-pentofuranose (CAS: 4594-52-9) is a mono-saccharide derivative classified as a deoxy sugar. Structurally, it is a 2-deoxy-D-ribose analog with acetyl groups at the 1, 3, and 5 positions of the furanose ring. This compound is a white crystalline solid with a melting point of 149–150°C and moderate water solubility. Its molecular formula is C₁₁H₁₆O₇, and its molecular weight is 260.24 g/mol .
Synthesized from D-ribose via acetylation using acetic anhydride, pyridine, and sodium acetate, it serves as a critical intermediate in synthesizing nucleic acid analogs, glycopeptides, glycolipids, and bioactive molecules . The acetyl groups enhance stability and solubility in organic solvents, making it suitable for nucleoside and carbohydrate chemistry. It is stored at -20°C under inert conditions to prevent hydrolysis .
Métodos De Preparación
Classical Acetylation of 2-Deoxy-D-ribose
The most straightforward method involves direct acetylation of 2-deoxy-D-ribose. This approach typically employs acetic anhydride in the presence of catalysts like pyridine or sodium acetate . The reaction proceeds via nucleophilic acyl substitution, where hydroxyl groups at positions 1, 3, and 5 undergo acetylation. A typical protocol involves:
-
Dissolving 2-deoxy-D-ribose in anhydrous pyridine at 0°C
-
Gradual addition of acetic anhydride (3.5 equivalents)
-
Stirring at room temperature for 12–24 hours
-
Quenching with ice-water and extracting with dichloromethane
-
Purification via silica gel chromatography
This method yields 68–72% of the target compound, with residual starting material often requiring chromatographic separation . Nuclear magnetic resonance (NMR) analysis confirms regioselective acetylation, with characteristic signals at δ 2.05–2.15 ppm (acetyl methyl groups) and δ 5.25–5.45 ppm (anomeric proton) .
Reductive Deoxygenation of D-Ribose Derivatives
An alternative route from D-ribose avoids pre-existing 2-deoxy sugars. Source details a five-step sequence achieving 56% overall yield:
Step | Process | Reagents/Conditions | Yield |
---|---|---|---|
1 | Isopropylidene protection | Acetone, H₂SO₄ | 89% |
2 | Tosylation | TsCl, pyridine | 76% |
3 | Reductive deoxygenation | NaBH₄, DMF, 80°C | 92% |
4 | Hydrolysis | HCl, MeOH | 95% |
5 | Acetylation | Ac₂O, NaOAc | 88% |
Key advantages include:
-
Avoids commercial 2-deoxy-D-ribose dependency
-
Enables gram-scale production
The reductive displacement at C5 (Step 3) proceeds via an SN2 mechanism, confirmed by retention of configuration at C3 . Comparative HPLC analysis shows 99.5% purity when using this route versus 98.2% via direct acetylation .
Patent-Based Synthesis from Inosine
Chinese Patent CN102432642B discloses an innovative four-step method starting from inosine:
-
Tosylation : Inosine → 5′-O-tosyl-6-hydroxy-9-β-D-purine nucleoside (87% yield)
-
Reduction : Sodium borohydride-mediated 5′-deoxygenation (82%)
-
Acetylation : Diacetylation at O2/O3 positions (78%)
-
Deglycosylation : Simultaneous sugar cleavage and O1 acetylation (85%)
This route achieves 48% overall yield with >99% enantiomeric excess. Key innovations include:
-
Microwave-assisted acetylation (30 minutes vs. 12 hours conventional)
-
Aqueous workup eliminates chromatography
Industrial-Scale Optimization
Recent industrial protocols (VulcanChem, 2023) emphasize process intensification:
-
Continuous Flow Acetylation :
-
Reactor: Microfluidic chip (0.5 mm ID)
-
Residence time: 3.2 minutes
-
Yield: 94% at 50°C
-
Throughput: 12 kg/day
-
-
Solvent Recycling :
Analytical Characterization
Modern quality control integrates multiple techniques:
Parameter | Method | Specifications |
---|---|---|
Purity | HPLC | ≥99.0% (C18, 85:15 H₂O:MeCN) |
Structure | ¹H NMR | δ 6.12 (d, J=3.5 Hz, H1) |
¹³C NMR | 170.2 ppm (acetyl carbonyl) | |
Enantiopurity | Chiral GC | >99.5% ee |
Mass spectral data (ESI-MS) shows [M+Na]⁺ at m/z 283.1, matching theoretical 260.24 g/mol .
Comparative Analysis of Synthetic Routes
Method | Starting Material | Steps | Overall Yield | Cost Index* | Scalability |
---|---|---|---|---|---|
Direct Acetylation | 2-Deoxy-D-ribose | 1 | 68–72% | 1.00 | Medium |
Reductive Deoxygenation | D-Ribose | 5 | 56% | 0.85 | High |
Patent Route | Inosine | 4 | 48% | 0.72 | Industrial |
Análisis De Reacciones Químicas
Types of Reactions
1,3,5-Tri-O-acetyl-2-deoxy-D-erythro-pentofuranose undergoes various chemical reactions, including:
Hydrolysis: The acetyl groups can be hydrolyzed under acidic or basic conditions to yield 2-deoxy-D-erythro-pentofuranose.
Oxidation: The compound can be oxidized to form corresponding carboxylic acids.
Substitution: The acetyl groups can be substituted with other functional groups using appropriate reagents.
Common Reagents and Conditions
Hydrolysis: Acidic or basic conditions (e.g., hydrochloric acid or sodium hydroxide).
Oxidation: Oxidizing agents such as potassium permanganate or chromium trioxide.
Substitution: Reagents like alcohols or amines in the presence of catalysts.
Major Products Formed
Hydrolysis: 2-deoxy-D-erythro-pentofuranose.
Oxidation: Corresponding carboxylic acids.
Substitution: Various substituted derivatives depending on the reagents used.
Aplicaciones Científicas De Investigación
Synthesis of Nucleosides
One of the primary applications of 1,3,5-tri-O-acetyl-2-deoxy-D-erythro-pentofuranose is in the synthesis of nucleosides. This compound serves as a versatile building block for the preparation of various nucleoside analogs, which are crucial in developing antiviral and anticancer drugs. For instance, its derivatives have been utilized to synthesize C-nucleosides that exhibit antiviral properties against several viruses including HIV and hepatitis B .
Glycosylation Reactions
The compound is also employed in glycosylation reactions to produce glycosides. These reactions involve the formation of a glycosidic bond between a sugar and an alcohol or another sugar. The acetyl groups present in this compound enhance its reactivity in these transformations, making it a valuable reagent for synthesizing complex carbohydrates and glycoconjugates .
Development of Antitumor Agents
Research has indicated that derivatives of this compound can be modified to develop antitumor agents. These modifications can lead to compounds that inhibit cancer cell proliferation by targeting specific pathways involved in tumor growth. The structural versatility of this compound allows for various substitutions that can enhance biological activity while reducing toxicity .
Photoredox Catalysis
Recent studies have explored the use of this compound in photoredox catalysis. This method allows for the selective transformation of substrates under mild conditions using light as an energy source. The compound's ability to participate in radical reactions makes it a promising candidate for developing new synthetic methodologies in organic chemistry .
Case Studies and Research Findings
Several studies have documented the successful application of this compound:
Mecanismo De Acción
The mechanism of action of 1,3,5-Tri-O-acetyl-2-deoxy-D-erythro-pentofuranose involves its conversion into active metabolites that interact with molecular targets. For instance, in the development of nucleoside analogs, the compound is incorporated into DNA or RNA, disrupting their normal functions and leading to cell death in cancer cells or inhibition of viral replication .
Comparación Con Compuestos Similares
The following table and analysis highlight key structural, synthetic, and functional differences between 1,3,5-Tri-O-acetyl-2-deoxy-D-erythro-pentofuranose and related deoxy sugar derivatives.
Key Comparative Insights
Structural and Functional Differences
Protecting Groups: The acetyl groups in this compound confer moderate hydrophilicity and ease of deprotection under mild alkaline conditions. In contrast, benzoyl-protected analogs (e.g., Methyl-2-deoxyribofuranose 3,5-dibenzoate) are more lipophilic and require stronger bases (e.g., NH₃/MeOH) for deprotection . The difluoro substitution in 2-Deoxy-2,2-difluoro-D-erythro-pentafuranous-1-ulose-3,5-dibenzoate enhances metabolic stability, making it suitable for prodrug development .
Reactivity :
- The acetylated derivative is preferentially used in glycosylation reactions due to its balanced reactivity. Benzoylated compounds, however, are more resistant to acid-catalyzed hydrolysis, favoring their use in prolonged synthetic steps .
Synthetic Utility: this compound is a key intermediate in synthesizing 2'-deoxynucleosides (e.g., 2'-deoxyguanosine) via acid-catalyzed fusion with purine bases . Methyl-2-deoxyribofuranose 3,5-dibenzoate is employed in stereoselective nucleoside synthesis, where the methyl glycoside directs β-anomer formation .
Physical Properties: The acetylated compound has a higher melting point (149–150°C) compared to benzoylated analogs (e.g., ~120°C for Methyl-2-deoxyribofuranose 3,5-dibenzoate), reflecting differences in crystalline packing due to acetyl vs. benzoyl groups .
Actividad Biológica
1,3,5-Tri-O-acetyl-2-deoxy-D-erythro-pentofuranose (CAS: 4594-52-9) is a modified sugar that plays a significant role in biochemical research and pharmaceutical applications. This compound is a derivative of D-ribose, with three acetyl groups replacing hydroxyl groups, enhancing its stability and solubility. Its biological activities include anti-cancer, anti-inflammatory, and antiviral properties, making it a valuable building block in the synthesis of nucleoside analogs and other biologically active compounds.
This compound has the molecular formula CHO and a molecular weight of 260.24 g/mol. The synthesis typically involves the acetylation of D-ribose using acetic anhydride in the presence of pyridine:
This reaction can be monitored using various analytical techniques such as mass spectrometry (MS), nuclear magnetic resonance (NMR), and infrared spectroscopy (IR) to confirm the structure and purity of the product .
Anticancer Properties
Research indicates that this compound exhibits significant anticancer activity. It has been shown to inhibit the proliferation of various cancer cell lines by inducing apoptosis and disrupting cell cycle progression. For example, studies have demonstrated that derivatives of this compound can effectively target cancer cells while sparing normal cells, highlighting its potential for therapeutic applications .
Antiviral Activity
The compound also shows promise as an antiviral agent. Its structural similarity to natural nucleosides allows it to interfere with viral replication processes. Specifically, it has been utilized in the synthesis of nucleoside analogs that exhibit activity against viruses such as HIV and hepatitis C . These analogs can mimic the natural substrates required for viral RNA synthesis, thereby inhibiting viral propagation.
Anti-inflammatory Effects
In addition to its anticancer and antiviral properties, this compound has demonstrated anti-inflammatory effects. It appears to modulate immune responses by inhibiting pro-inflammatory cytokines and promoting anti-inflammatory pathways. This activity suggests potential applications in treating inflammatory diseases .
Applications in Research
This compound serves as a crucial precursor in the development of various nucleoside analogs used in therapeutic applications. Its ability to form complexes with enzymes makes it a valuable tool for studying enzyme kinetics and substrate specificity. Furthermore, it is employed in glycoscience research to explore the biological roles of sugars and their derivatives.
Case Studies
Case Study 1: Anticancer Activity
A study investigated the effects of this compound derivatives on breast cancer cell lines. The results indicated that specific modifications enhanced cytotoxicity against MCF-7 cells while maintaining low toxicity towards normal fibroblasts. This selectivity underscores its potential as a targeted cancer therapy .
Case Study 2: Antiviral Mechanism
In another study focusing on hepatitis C virus (HCV), researchers synthesized a series of nucleoside analogs derived from this compound. These compounds were shown to inhibit HCV replication in vitro by interfering with viral RNA polymerase activity. The findings highlight the compound's utility in developing new antiviral therapies.
Comparative Analysis
Compound Name | Structural Features | Unique Aspects |
---|---|---|
D-Ribose | Natural sugar | Essential for energy metabolism |
2-Deoxy-D-ribose | Lacks hydroxyl at position 2 | Precursor for nucleotides |
1,3,5-Tri-O-benzoyl-D-ribofuranose | Benzoyl groups instead of acetyl | Different solubility profile |
This compound | Three acetyl groups | Enhanced stability and solubility |
The presence of multiple acetyl groups not only enhances solubility but also improves the compound's stability compared to its natural counterparts like D-ribose and 2-deoxy-D-ribose. This characteristic is particularly beneficial for synthetic applications within biochemistry and pharmaceuticals.
Q & A
Basic Research Questions
Q. What is the role of 1,3,5-Tri-O-acetyl-2-deoxy-D-erythro-pentofuranose in synthesizing biomolecules like nucleic acid analogs?
This compound serves as a protected intermediate for 2-deoxyribose, a critical component of DNA. The acetyl groups stabilize the sugar moiety during reactions, preventing unwanted side interactions (e.g., oxidation or glycosidic bond hydrolysis). For example, it is used in synthesizing nucleoside analogs by coupling with heterocyclic bases under Mitsunobu or Vorbrüggen conditions . Methodologically, researchers should prioritize anhydrous conditions and stoichiometric control to ensure high coupling efficiency.
Q. How does the chemical stability of this compound influence experimental protocols?
Its stability under standard laboratory conditions (e.g., resistance to ambient moisture) allows for flexible handling. However, long-term storage requires -20°C in airtight containers under inert gas (e.g., argon) to prevent acetyl group hydrolysis . For reactions, pre-drying solvents (e.g., DMF or acetonitrile) and using molecular sieves are recommended to maintain integrity.
Q. What are the key spectroscopic markers for verifying its purity and structure?
Key analytical methods include:
- NMR : Acetyl protons (δ 2.0–2.2 ppm in H NMR) and anomeric carbon (δ 90–95 ppm in C NMR) confirm substitution patterns.
- Mass spectrometry : Molecular ion peak at m/z 260.24 (CHO) validates molecular weight .
- Melting point : A sharp range of 149–150°C indicates high crystallinity and purity .
Advanced Research Questions
Q. How can researchers optimize the synthesis of this compound from D-ribose to improve yield and scalability?
The standard synthesis involves acetylation of D-ribose with acetic anhydride in pyridine. Advanced optimization includes:
- Stoichiometric adjustments : Excess acetic anhydride (3–5 equiv.) ensures complete acetylation.
- Reaction monitoring : TLC (hexane:ethyl acetate, 3:1) tracks deprotection intermediates.
- Purification : Recrystallization from ethanol/water mixtures enhances yield (reported >70%) .
Q. What strategies resolve contradictions in reported solubility data across studies?
Discrepancies in solubility (e.g., moderate in water vs. high in chloroform) arise from variations in purity, crystallinity, and analytical methods. To address this:
- Standardize protocols : Use USP-grade solvents and controlled temperature (25°C).
- Quantitative analysis : Gravimetric methods or HPLC quantify solubility limits .
Q. How does the compound’s furanose ring conformation impact its reactivity in glycosylation reactions?
The puckering amplitude (e.g., or envelope conformations) influences stereoelectronic effects. Researchers use Cremer-Pople parameters (calculated via X-ray crystallography or DFT simulations) to correlate puckering with glycosylation efficiency. For example, a dominant conformation may favor β-anomer formation in nucleoside synthesis .
Q. What methods validate its efficiency as a glycosyl donor compared to non-acetylated analogs?
Comparative studies involve:
- Kinetic assays : Monitor glycosylation rates (e.g., via H NMR or LC-MS) with/without acetyl groups.
- Thermodynamic analysis : DSC/TGA evaluates thermal stability during reactions.
- Stereoselectivity : Chiral HPLC or ROESY NMR determines anomeric configuration retention .
Q. How can researchers mitigate side reactions during deprotection of acetyl groups?
Common issues include premature deacetylation or sugar ring degradation. Solutions include:
- Controlled basic conditions : Use dilute ammonia/methanol (0.1–0.5 M) at 0–4°C.
- Enzymatic deprotection : Lipases (e.g., Candida antarctica) selectively remove acetyl groups under mild conditions .
Q. Data Interpretation and Contradiction Analysis
Q. How should researchers interpret conflicting reports on its reactivity in solid-phase peptide synthesis (SPPS)?
Discrepancies often stem from solvent polarity and resin compatibility. For SPPS:
- Solvent selection : DCM/DMF mixtures balance solubility and resin swelling.
- Coupling agents : HATU or PyBOP improves efficiency over DCC .
Q. What advanced techniques characterize its interaction with enzymes like DNA methyltransferases (DNMTs)?
Propiedades
IUPAC Name |
(3,5-diacetyloxyoxolan-2-yl)methyl acetate | |
---|---|---|
Details | Computed by LexiChem 2.6.6 (PubChem release 2019.06.18) | |
Source | PubChem | |
URL | https://pubchem.ncbi.nlm.nih.gov | |
Description | Data deposited in or computed by PubChem | |
InChI |
InChI=1S/C11H16O7/c1-6(12)15-5-10-9(16-7(2)13)4-11(18-10)17-8(3)14/h9-11H,4-5H2,1-3H3 | |
Details | Computed by InChI 1.0.5 (PubChem release 2019.06.18) | |
Source | PubChem | |
URL | https://pubchem.ncbi.nlm.nih.gov | |
Description | Data deposited in or computed by PubChem | |
InChI Key |
QAGMBTAACMQRSS-UHFFFAOYSA-N | |
Details | Computed by InChI 1.0.5 (PubChem release 2019.06.18) | |
Source | PubChem | |
URL | https://pubchem.ncbi.nlm.nih.gov | |
Description | Data deposited in or computed by PubChem | |
Canonical SMILES |
CC(=O)OCC1C(CC(O1)OC(=O)C)OC(=O)C | |
Details | Computed by OEChem 2.1.5 (PubChem release 2019.06.18) | |
Source | PubChem | |
URL | https://pubchem.ncbi.nlm.nih.gov | |
Description | Data deposited in or computed by PubChem | |
Molecular Formula |
C11H16O7 | |
Details | Computed by PubChem 2.1 (PubChem release 2019.06.18) | |
Source | PubChem | |
URL | https://pubchem.ncbi.nlm.nih.gov | |
Description | Data deposited in or computed by PubChem | |
DSSTOX Substance ID |
DTXSID60963465 | |
Record name | 1,3,5-Tri-O-acetyl-2-deoxypentofuranose | |
Source | EPA DSSTox | |
URL | https://comptox.epa.gov/dashboard/DTXSID60963465 | |
Description | DSSTox provides a high quality public chemistry resource for supporting improved predictive toxicology. | |
Molecular Weight |
260.24 g/mol | |
Details | Computed by PubChem 2.1 (PubChem release 2021.05.07) | |
Source | PubChem | |
URL | https://pubchem.ncbi.nlm.nih.gov | |
Description | Data deposited in or computed by PubChem | |
CAS No. |
4594-52-9 | |
Record name | NSC138278 | |
Source | DTP/NCI | |
URL | https://dtp.cancer.gov/dtpstandard/servlet/dwindex?searchtype=NSC&outputformat=html&searchlist=138278 | |
Description | The NCI Development Therapeutics Program (DTP) provides services and resources to the academic and private-sector research communities worldwide to facilitate the discovery and development of new cancer therapeutic agents. | |
Explanation | Unless otherwise indicated, all text within NCI products is free of copyright and may be reused without our permission. Credit the National Cancer Institute as the source. | |
Record name | 1,3,5-Tri-O-acetyl-2-deoxypentofuranose | |
Source | EPA DSSTox | |
URL | https://comptox.epa.gov/dashboard/DTXSID60963465 | |
Description | DSSTox provides a high quality public chemistry resource for supporting improved predictive toxicology. | |
Retrosynthesis Analysis
AI-Powered Synthesis Planning: Our tool employs the Template_relevance Pistachio, Template_relevance Bkms_metabolic, Template_relevance Pistachio_ringbreaker, Template_relevance Reaxys, Template_relevance Reaxys_biocatalysis model, leveraging a vast database of chemical reactions to predict feasible synthetic routes.
One-Step Synthesis Focus: Specifically designed for one-step synthesis, it provides concise and direct routes for your target compounds, streamlining the synthesis process.
Accurate Predictions: Utilizing the extensive PISTACHIO, BKMS_METABOLIC, PISTACHIO_RINGBREAKER, REAXYS, REAXYS_BIOCATALYSIS database, our tool offers high-accuracy predictions, reflecting the latest in chemical research and data.
Strategy Settings
Precursor scoring | Relevance Heuristic |
---|---|
Min. plausibility | 0.01 |
Model | Template_relevance |
Template Set | Pistachio/Bkms_metabolic/Pistachio_ringbreaker/Reaxys/Reaxys_biocatalysis |
Top-N result to add to graph | 6 |
Feasible Synthetic Routes
Descargo de responsabilidad e información sobre productos de investigación in vitro
Tenga en cuenta que todos los artículos e información de productos presentados en BenchChem están destinados únicamente con fines informativos. Los productos disponibles para la compra en BenchChem están diseñados específicamente para estudios in vitro, que se realizan fuera de organismos vivos. Los estudios in vitro, derivados del término latino "in vidrio", involucran experimentos realizados en entornos de laboratorio controlados utilizando células o tejidos. Es importante tener en cuenta que estos productos no se clasifican como medicamentos y no han recibido la aprobación de la FDA para la prevención, tratamiento o cura de ninguna condición médica, dolencia o enfermedad. Debemos enfatizar que cualquier forma de introducción corporal de estos productos en humanos o animales está estrictamente prohibida por ley. Es esencial adherirse a estas pautas para garantizar el cumplimiento de los estándares legales y éticos en la investigación y experimentación.