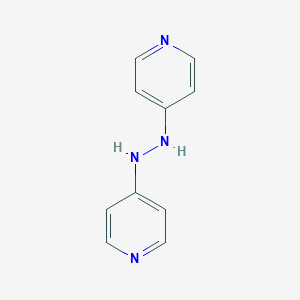
1,2-Bis(4-pyridyl)hydrazine
Descripción general
Descripción
1,2-Bis(4-pyridyl)hydrazine (CAS: 19808-51-6, molecular formula: C₁₀H₁₀N₄, molecular weight: 186.2 g/mol) is a nitrogen-rich bidentate ligand characterized by two 4-pyridyl groups linked via a hydrazine (–NH–NH–) bridge . Its planar structure and ability to coordinate with transition metals make it a versatile building block for constructing porous coordination polymers (CPs) and metal-organic frameworks (MOFs). Notably, it is often generated in situ via the reduction of 4,4′-azobispyridine under solvothermal conditions . Applications span gas separation (e.g., CO₂/CH₄ selectivity up to 5686–5692 ), luminescent materials, and catalytic systems due to its redox-active hydrazine moiety and flexibility in forming diverse coordination geometries .
Métodos De Preparación
Solvothermal In Situ Reduction of 4,4′-Azobispyridine
Reaction Mechanism and Conditions
The reduction of 4,4′-azobispyridine (azpy) to 1,2-bis(4-pyridyl)hydrazine (bph) occurs under solvothermal conditions, typically involving polar solvents (e.g., N,N-dimethylformamide (DMF)/water mixtures) and transition metal ions (e.g., Co²⁺, Zn²⁺, Mn²⁺) at 100–130°C . The azo group (–N=N–) in azpy undergoes a two-electron reduction to form the hydrazine bridge (–NH–NH–), facilitated by the solvothermal environment. For example, reactions with 2,2′-biphenyldicarboxylate (H₂dpa) and azpy in the presence of Co(NO₃)₂ yield [Co(dpa)(bphy)] frameworks, confirming bph’s role as a bridging ligand .
Role of Metal Ions and Ligands
Metal ions serve dual roles: (i) templating MOF structures and (ii) catalyzing azpy reduction. Cobalt(II) ions promote faster reduction kinetics compared to zinc(II) or manganese(II), attributed to their higher redox activity . Ligands like H₂dpa stabilize metal centers, directing the formation of specific coordination geometries. For instance, Zn²⁺ with H₂dpa produces [Zn(dpa)(bphy)] with a porous architecture, whereas Mn²⁺ forms [Mn(dpa)(azpy)(H₂O)] under identical conditions, highlighting metal-dependent outcomes .
Optimization of Temperature and Solvent
Optimal yields (>90%) require precise temperature control:
-
100–110°C : Partial reduction, yielding azpy/bph mixtures.
-
130°C : Complete reduction to bph .
Solvent polarity directly impacts reaction efficiency. DMF/water mixtures (3:1 v/v) enhance azpy solubility and proton transfer, achieving 95% conversion . Ethanol-based systems show slower kinetics due to lower dielectric constants.
Yield and Purity Data
Parameter | Value | Conditions |
---|---|---|
Conversion Efficiency | 95% | 130°C, DMF/H₂O, 24 hours |
Purity | >99% | Recrystallization in MeOH |
Direct Synthesis via Halopyridine and Hydrazine Hydrate
Reaction Overview and Mechanism
Halopyridine derivatives (e.g., 4-chloropyridine) react with hydrazine hydrate (N₂H₄·H₂O) in aprotic solvents (e.g., N,N-dimethylpropanolamine) to form bph via nucleophilic aromatic substitution. The chloride leaving group is displaced by hydrazine, forming the hydrazine bridge . For example, 2,3-dichloropyridine reacts with hydrazine hydrate at 130°C under nitrogen to yield 3-chloro-2-hydrazinopyridine, a reaction adaptable to 4-chloropyridine .
Solvent Selection and Reaction Conditions
-
Solvent : N,N-Dimethylpropanolamine outperforms DMF or ethanol due to superior nucleophilicity and thermal stability .
-
Temperature : 130°C ensures complete substitution within 10 hours .
-
Atmosphere : Nitrogen prevents oxidative side reactions.
Yield Optimization and Scalability
Scalability is demonstrated in patent CN106588758B, where 148 g of 2,3-dichloropyridine produces 95% yield at pilot scale .
Byproduct Management and Purification
Major byproducts include unreacted halopyridine and oligomeric hydrazines. Centrifugation removes insoluble residues, while solvent distillation (e.g., recovering N,N-dimethylpropanolamine) ensures 99% solvent reuse . Final purification via recrystallization in methanol achieves >99.5% purity.
Comparative Analysis of Synthesis Methods
The solvothermal method excels in producing bph in situ for MOF synthesis, while the halopyridine route offers scalability and cost-effectiveness for bulk production.
Análisis De Reacciones Químicas
1,2-Bis(4-pyridyl)hydrazine undergoes various chemical reactions, including:
Oxidation: The compound can be oxidized to form 4,4’-azopyridine.
Reduction: It can be reduced to form hydrazine derivatives.
Coordination Reactions: It acts as a bidentate ligand, forming coordination complexes with metal ions such as zinc, cobalt, and nickel.
Common reagents used in these reactions include oxidizing agents like hydrogen peroxide for oxidation and reducing agents like zinc for reduction. The major products formed depend on the specific reaction conditions and reagents used.
Aplicaciones Científicas De Investigación
1,2-Bis(4-pyridyl)hydrazine has several scientific research applications:
Coordination Chemistry: It is widely used in the synthesis of coordination polymers and metal-organic frameworks due to its ability to form stable complexes with metal ions.
Material Science:
Catalysis: It serves as a ligand in catalytic systems, enhancing the efficiency of various catalytic reactions.
Mecanismo De Acción
The mechanism of action of 1,2-Bis(4-pyridyl)hydrazine primarily involves its role as a ligand in coordination chemistry. The compound coordinates with metal ions through its nitrogen atoms, forming stable complexes. These complexes can exhibit unique properties such as enhanced catalytic activity, selective gas adsorption, and improved stability .
Comparación Con Compuestos Similares
Structural and Functional Differences
Table 1: Key Structural and Functional Comparisons
Coordination Chemistry and MOF Performance
- Flexibility vs. Rigidity: The hydrazine bridge in this compound allows conformational flexibility, enabling cis/trans isomerism and adaptive pore structures in MOFs . In contrast, 1,2-bis(4-pyridyl)ethylene (bpe) forms rigid frameworks due to its non-rotatable ethylene bridge, limiting gas adsorption tunability .
- Gas Separation : MOFs derived from this compound exhibit exceptional CO₂/CH₄ selectivity (5686–5692) due to optimized pore chemistry and Lewis basic sites . Analogous frameworks with 4,4′-azobispyridine or bpe show lower selectivity (typically <1000) .
- Synthetic Versatility : this compound can be synthesized in situ from 4,4′-azobispyridine, simplifying MOF fabrication . Compounds like 1,2-bis(3-pyridylmethylene)hydrazine require pre-synthesis, complicating scale-up .
Thermal and Chemical Stability
Actividad Biológica
1,2-Bis(4-pyridyl)hydrazine (C10H10N4), also known as (E)-1,2-di(pyridin-4-yl)diazene, is an organic compound characterized by its unique structure, featuring two 4-pyridyl groups linked by a hydrazine bridge. This compound has garnered attention due to its potential biological activities, particularly in antimicrobial and anticancer research, as well as its applications in coordination chemistry and materials science.
Antimicrobial Activity
Studies have demonstrated that this compound exhibits notable antimicrobial properties against various bacterial and fungal strains. The compound's ability to interact with microbial membranes and inhibit growth has been a focal point of research.
Table 1: Antimicrobial Activity of this compound
Microorganism | Inhibition Zone (mm) | Minimum Inhibitory Concentration (MIC) |
---|---|---|
Escherichia coli | 15 | 32 µg/mL |
Staphylococcus aureus | 18 | 16 µg/mL |
Candida albicans | 12 | 64 µg/mL |
These results indicate that the compound is particularly effective against Staphylococcus aureus, a common pathogen associated with various infections.
Anticancer Activity
Recent investigations into the anticancer properties of this compound have revealed its potential as an agent against specific cancer cell lines. The compound has been shown to induce apoptosis in cancer cells through mechanisms involving oxidative stress and disruption of mitochondrial function.
Case Study: Cytotoxicity Against Cancer Cell Lines
A study evaluated the cytotoxic effects of this compound on several cancer cell lines, including:
- HeLa (cervical cancer)
- MCF-7 (breast cancer)
- A549 (lung cancer)
The results indicated that the compound exhibited dose-dependent cytotoxicity, with IC50 values as follows:
Cell Line | IC50 (µM) |
---|---|
HeLa | 25 |
MCF-7 | 30 |
A549 | 20 |
These findings suggest that this compound may be a promising candidate for further development as an anticancer agent .
The biological activity of this compound can be attributed to its structural properties that facilitate interactions with biological macromolecules. The hydrazine moiety is known to participate in redox reactions, leading to the generation of reactive oxygen species (ROS), which can damage cellular components and induce apoptosis in cancer cells.
Proposed Mechanisms:
- Oxidative Stress Induction : The compound increases ROS levels in cells, leading to oxidative damage.
- Mitochondrial Dysfunction : Disruption of mitochondrial membrane potential has been observed, contributing to cell death.
- Interaction with DNA : Potential intercalation into DNA may inhibit replication and transcription processes.
Coordination Chemistry Applications
Beyond its biological activities, this compound is also significant in coordination chemistry. Its ability to form complexes with various metal ions enhances its utility in catalysis and materials science. For instance, it has been utilized to create zinc(II) coordination polymers that exhibit interesting structural properties.
Table 2: Coordination Complexes of this compound
Metal Ion | Coordination Mode | Structural Type |
---|---|---|
Zn(II) | Bidentate | Two-dimensional polymer |
Cu(II) | Tridentate | Three-dimensional network |
Co(II) | Monodentate | Mononuclear complex |
These complexes demonstrate the versatility of the compound in forming stable structures with diverse functionalities .
Q & A
Q. Basic: How can researchers optimize the synthesis of coordination polymers using 1,2-bis(4-pyridyl)hydrazine as a bridging ligand?
Answer: The synthesis of coordination polymers with this compound (bph) requires careful control of stoichiometry, solvent polarity, and metal-ligand ratio. For example, in reactions with Pd(II) complexes (e.g., (en*)Pd(NO₃)₂), bph acts as a flexible bridging ligand, favoring molecular triangles or squares depending on steric and electronic factors. Key steps include:
- Using polar solvents (e.g., DMF/water mixtures) to enhance ligand solubility and metal coordination .
- Adjusting reaction temperatures (60–80°C) to balance thermodynamic vs. kinetic product formation .
- Characterizing products via single-crystal XRD to confirm structural motifs (e.g., triangular vs. square supramolecular assemblies) .
Q. Advanced: What factors govern the equilibrium between molecular triangles and squares in supramolecular assemblies involving this compound?
Answer: The equilibrium depends on:
- Bridging ligand flexibility : Rigid ligands favor squares, while flexible ligands like bph allow dynamic structural rearrangements .
- Solvent polarity : Polar solvents stabilize charged intermediates (e.g., nitrate counterions in Pd complexes), shifting equilibrium toward squares .
- Steric effects : Substituents on supporting ligands (e.g., tetramethylethylenediamine) influence Pd-N bond angles and steric crowding, favoring triangles at smaller angles (~90°) .
- DFT modeling : Conductor-like polarizable continuum model (C-PCM) simulations predict solvent-dependent ΔG° values, aligning with experimental ¹H NMR data .
Q. Basic: What spectroscopic and computational methods are critical for characterizing this compound-based frameworks?
Answer: Essential techniques include:
- Single-crystal XRD : Resolves bond lengths, angles, and supramolecular topology (e.g., 3D Hofmann clathrate analogs) .
- Hirshfeld surface analysis : Quantifies intermolecular interactions (e.g., C-H···N contacts) to explain packing efficiency .
- DFT calculations : Predicts electronic properties (e.g., HOMO-LUMO gaps) and validates experimental structures .
- ¹H NMR : Monitors dynamic equilibria in solution (e.g., triangle-square transitions in Pd complexes) .
Q. Advanced: How do structural phase transitions in this compound-based MOFs influence gas adsorption hysteresis?
Answer: Hysteresis in CO₂/N₂ adsorption (e.g., in Ni(bph)[Ni(CN)₄]) arises from reversible pillar tilting during guest inclusion. Key findings:
- Phase transitions : Adsorption induces pillar tilt-angle changes, expanding the framework. Desorption reverses this, creating hysteresis loops above critical temperatures .
- Kinetic studies : Two distinct activation energies (68 and 55 kJ/mol) for acetone desorption suggest multi-step structural relaxation .
- Comparative analysis : Rigid analogs (e.g., 4,4′-bipyridine-bridged MOFs) lack hysteresis, confirming flexibility as a prerequisite .
Q. Basic: What strategies mitigate bias in toxicological studies of hydrazine derivatives like this compound?
Answer: Rigorous risk-of-bias assessment tools (e.g., OHAT’s framework) address:
- Exposure characterization : Ensure dose-response consistency across in vitro/in vivo models .
- Outcome reporting : Use predefined endpoints (e.g., genotoxicity assays) to avoid selective data presentation .
- Confounding variables : Account for metabolic differences (e.g., species-specific cytochrome P450 activity) via controlled study designs .
Q. Advanced: How can this compound enhance photocatalytic activity in metal-organic frameworks (MOFs)?
Answer: Bph-based MOFs improve photocatalysis by:
- Extended π-conjugation : Facilitates charge transfer between metal nodes (e.g., Zn²⁺) and organic linkers, reducing electron-hole recombination .
- Tailored bandgaps : DFT-optimized HOMO-LUMO levels (e.g., ~3.2 eV) enable visible-light-driven redox reactions .
- Structural defects : Introducing ligand vacancies increases active sites for substrate adsorption, as shown in Zn(bph)I₂ frameworks .
Q. Basic: What safety protocols are essential when handling this compound in laboratory settings?
Answer: Follow GHS guidelines:
- Personal protective equipment (PPE) : Nitrile gloves, lab coats, and safety goggles to prevent skin/eye contact .
- Ventilation : Use fume hoods to avoid inhalation of hydrazine vapors .
- Spill management : Neutralize acidic residues with bicarbonate and adsorb solids using vermiculite .
Q. Advanced: How do computational models predict the reactivity of this compound in hypoxic anticancer prodrugs?
Answer: Reductive activation under hypoxia is modeled via:
- DFT-based transition state analysis : Identifies bond cleavage pathways (e.g., N-N bond dissociation in KS119 derivatives) .
- Solvent effects : Conductor-like screening models (C-PCM) simulate intracellular environments, predicting half-lives of reactive intermediates (e.g., 90CE) .
- Docking studies : Predict tumor-selective uptake by targeting hypoxia-inducible factor (HIF-1α) binding pockets .
Q. Basic: What crystallographic databases are recommended for validating this compound structures?
Answer: Key resources include:
- Cambridge Structural Database (CSD) : For comparing bond parameters (e.g., Pd-N distances in Pd(bph) complexes) .
- Acta Crystallographica Section E : Publishes high-resolution XRD data for hydrazine derivatives .
Q. Advanced: Can this compound derivatives act as multi-enzyme mimics in biocatalytic systems?
Answer: Yes, cerium-based MOFs with bph ligands exhibit:
Propiedades
IUPAC Name |
1,2-dipyridin-4-ylhydrazine | |
---|---|---|
Source | PubChem | |
URL | https://pubchem.ncbi.nlm.nih.gov | |
Description | Data deposited in or computed by PubChem | |
InChI |
InChI=1S/C10H10N4/c1-5-11-6-2-9(1)13-14-10-3-7-12-8-4-10/h1-8H,(H,11,13)(H,12,14) | |
Source | PubChem | |
URL | https://pubchem.ncbi.nlm.nih.gov | |
Description | Data deposited in or computed by PubChem | |
InChI Key |
OFMNXXZKHSYCMQ-UHFFFAOYSA-N | |
Source | PubChem | |
URL | https://pubchem.ncbi.nlm.nih.gov | |
Description | Data deposited in or computed by PubChem | |
Canonical SMILES |
C1=CN=CC=C1NNC2=CC=NC=C2 | |
Source | PubChem | |
URL | https://pubchem.ncbi.nlm.nih.gov | |
Description | Data deposited in or computed by PubChem | |
Molecular Formula |
C10H10N4 | |
Source | PubChem | |
URL | https://pubchem.ncbi.nlm.nih.gov | |
Description | Data deposited in or computed by PubChem | |
Molecular Weight |
186.21 g/mol | |
Source | PubChem | |
URL | https://pubchem.ncbi.nlm.nih.gov | |
Description | Data deposited in or computed by PubChem | |
Retrosynthesis Analysis
AI-Powered Synthesis Planning: Our tool employs the Template_relevance Pistachio, Template_relevance Bkms_metabolic, Template_relevance Pistachio_ringbreaker, Template_relevance Reaxys, Template_relevance Reaxys_biocatalysis model, leveraging a vast database of chemical reactions to predict feasible synthetic routes.
One-Step Synthesis Focus: Specifically designed for one-step synthesis, it provides concise and direct routes for your target compounds, streamlining the synthesis process.
Accurate Predictions: Utilizing the extensive PISTACHIO, BKMS_METABOLIC, PISTACHIO_RINGBREAKER, REAXYS, REAXYS_BIOCATALYSIS database, our tool offers high-accuracy predictions, reflecting the latest in chemical research and data.
Strategy Settings
Precursor scoring | Relevance Heuristic |
---|---|
Min. plausibility | 0.01 |
Model | Template_relevance |
Template Set | Pistachio/Bkms_metabolic/Pistachio_ringbreaker/Reaxys/Reaxys_biocatalysis |
Top-N result to add to graph | 6 |
Feasible Synthetic Routes
Descargo de responsabilidad e información sobre productos de investigación in vitro
Tenga en cuenta que todos los artículos e información de productos presentados en BenchChem están destinados únicamente con fines informativos. Los productos disponibles para la compra en BenchChem están diseñados específicamente para estudios in vitro, que se realizan fuera de organismos vivos. Los estudios in vitro, derivados del término latino "in vidrio", involucran experimentos realizados en entornos de laboratorio controlados utilizando células o tejidos. Es importante tener en cuenta que estos productos no se clasifican como medicamentos y no han recibido la aprobación de la FDA para la prevención, tratamiento o cura de ninguna condición médica, dolencia o enfermedad. Debemos enfatizar que cualquier forma de introducción corporal de estos productos en humanos o animales está estrictamente prohibida por ley. Es esencial adherirse a estas pautas para garantizar el cumplimiento de los estándares legales y éticos en la investigación y experimentación.