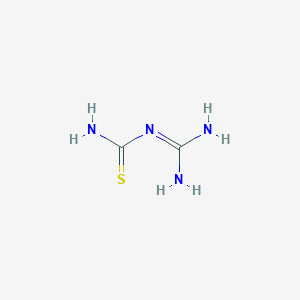
Guaniltiourea
Descripción general
Descripción
May protect against hypoxic damage; proposed for treatment of shock due to trauma or blood loss; also stimulates paretic gastrointestinal system.
Aplicaciones Científicas De Investigación
Aplicaciones Industriales
La Guaniltiourea tiene amplias aplicaciones industriales . En conjunto con el disulfuro de mercaptobenzotiazilo, se utiliza como acelerador en la vulcanización de azufre del caucho natural .
Síntesis Química
En la síntesis química, su esqueleto molecular especial y su capacidad de enlace de hidrógeno se han utilizado para sintetizar compuestos supramoleculares enjaulados con aniones .
Aplicaciones Médicas
La this compound encuentra las aplicaciones más importantes en el campo de la medicina . Es un estimulador conocido del peristaltismo intestinal y se ha aplicado clínicamente para tratar la paresia intestinal en la peritonitis .
Inmunoestimulante e Inhibidor de Células Tumorales
La this compound ha mostrado un uso prometedor en ensayos clínicos como inmunoestimulante e inhibidor de células tumorales .
Agentes Radioprotectores
Los compuestos orgánicos que incorporan unidades tioureido y guanidino son útiles como agentes radioprotectores . De hecho, se ha demostrado que la this compound y sus derivados dan una tasa de supervivencia del 66% cuando se someten a dosis de radiación potencialmente letales .
Química Supramolecular
En el campo de la química supramolecular, la investigación que involucra this compound se centra principalmente en su coordinación a metales de transición, generando así contenedores moleculares que pueden mejorar su acidez de Lewis o introducir propiedades magnéticas, electroquímicas y/o luminiscentes en las moléculas huésped
Mecanismo De Acción
Target of Action
Guanylthiourea (GTU) has been identified as a potential inhibitor of the enzyme furin , a subtilisin-like proprotein convertase . Furin is a promising therapeutic target for several viral manifestations . GTU derivatives have also been identified as potential anti-malarial agents, showing activity against Plasmodium falciparum .
Mode of Action
Molecular docking and dynamics studies suggest that GTU and its derivatives interact with furin’s catalytic triad . The interaction is influenced by substituent-guided tailored interactions . For instance, single arm GTU substitution influences the interactions with Asp153, His194, and Ser368 .
Biochemical Pathways
The interaction of gtu with furin’s catalytic triad suggests that it may interfere with the enzyme’s ability to process and activate proteins, thereby potentially inhibiting the life cycle of certain viruses .
Pharmacokinetics
The design of potential furin inhibitors like gtu involves a detailed mechanistic analysis of specific molecular interactions . This suggests that the ADME properties of GTU and its impact on bioavailability are considered during the design process.
Result of Action
The result of GTU’s action is the potential inhibition of furin, which could lead to the systemic development of specific and potent antivirals . Additionally, GTU derivatives have shown in vivo anti-malarial activity against experimental rodent malaria .
Action Environment
The action environment of GTU can influence its efficacy and stability. For instance, the synthesis of GTU involves a reaction with hydrogen sulfide at specific temperatures . This suggests that the stability and efficacy of GTU could be influenced by factors such as temperature and pH.
Análisis Bioquímico
Biochemical Properties
In biochemical reactions, Guanylthiourea derivatives interact with various enzymes, proteins, and other biomolecules. These interactions play a crucial role in its anti-malarial activity
Cellular Effects
Guanylthiourea derivatives have been shown to have significant effects on various types of cells and cellular processes. They influence cell function, including impacts on cell signaling pathways, gene expression, and cellular metabolism .
Molecular Mechanism
The molecular mechanism of action of Guanylthiourea is complex and multifaceted. It exerts its effects at the molecular level, including binding interactions with biomolecules, enzyme inhibition or activation, and changes in gene expression .
Temporal Effects in Laboratory Settings
In laboratory settings, the effects of Guanylthiourea change over time. Information on the product’s stability, degradation, and any long-term effects on cellular function observed in in vitro or in vivo studies is currently being researched .
Dosage Effects in Animal Models
The effects of Guanylthiourea vary with different dosages in animal models. Studies have observed threshold effects, as well as toxic or adverse effects at high doses .
Metabolic Pathways
Guanylthiourea is involved in various metabolic pathways. It interacts with several enzymes or cofactors, and can affect metabolic flux or metabolite levels .
Transport and Distribution
The transport and distribution of Guanylthiourea within cells and tissues are complex processes that involve various transporters or binding proteins. It can also affect its localization or accumulation .
Subcellular Localization
The subcellular localization of Guanylthiourea and its effects on its activity or function are areas of active research. This could include any targeting signals or post-translational modifications that direct it to specific compartments or organelles .
Actividad Biológica
Guanylthiourea (GTU) is a compound that has garnered attention in various fields of biological research due to its diverse biological activities. This article explores the biological properties of GTU, including its mechanisms of action, applications in pharmacology, and findings from recent studies.
Chemical Structure and Properties
Guanylthiourea is derived from dicyandiamide and consists of a thiourea functional group. It exhibits two primary tautomeric forms: thione and thiol. The stability and reactivity of these forms are influenced by intramolecular hydrogen bonding and the conjugation of pi-electrons, which play a significant role in its biological activity .
1. Antimalarial Activity
Recent studies have identified GTU as an important pharmacophore in the development of antimalarial agents. It has been incorporated into the structure of 4-aminoquinoline derivatives, which have shown promising antimalarial activity against Plasmodium falciparum. In vitro evaluations revealed that several synthesized compounds containing GTU exhibited effective inhibition with IC50 values ranging from 0.43 to 8.04 μM against different strains of P. falciparum (PfD6 and PfW2) .
2. Hypoglycemic Effects
GTU has been studied for its potential as a hypoglycemic agent. It acts by enhancing insulin sensitivity and promoting glucose uptake in peripheral tissues. This effect is particularly beneficial for managing conditions like type 2 diabetes mellitus. The mechanism involves the modulation of glucose transporters and insulin signaling pathways .
3. Antitumor Activity
Research indicates that GTU derivatives possess antitumor properties, inhibiting the proliferation of various cancer cell lines. The compound's ability to interfere with cellular signaling pathways involved in tumor growth has been documented, suggesting potential applications in cancer therapy .
4. Immunomodulatory Effects
GTU has also shown immunostimulant properties, enhancing immune responses in experimental models. This activity may be attributed to its influence on cytokine production and immune cell activation, making it a candidate for further exploration in immunotherapy .
The mechanisms underlying the biological activities of GTU are multifaceted:
- Dihydrofolate Reductase Inhibition : GTU has been shown to inhibit dihydrofolate reductase (DHFR), an enzyme critical for DNA synthesis and repair, which is particularly relevant in antimalarial and anticancer contexts .
- Reactive Oxygen Species Modulation : Some studies suggest that GTU may modulate oxidative stress levels within cells, contributing to its cytotoxic effects against tumor cells .
Study on Antimalarial Activity
In a study evaluating the antimalarial potential of GTU-containing compounds, researchers synthesized nine derivatives through molecular docking techniques targeting PfDHFR. The most active compound demonstrated significant binding interactions with the enzyme, leading to effective inhibition of P. falciparum growth in vitro .
Hypoglycemic Activity Assessment
A clinical study assessed the hypoglycemic effects of GTU in diabetic animal models. Results indicated a significant reduction in blood glucose levels following administration of GTU, highlighting its potential therapeutic role in diabetes management .
Propiedades
IUPAC Name |
diaminomethylidenethiourea | |
---|---|---|
Source | PubChem | |
URL | https://pubchem.ncbi.nlm.nih.gov | |
Description | Data deposited in or computed by PubChem | |
InChI |
InChI=1S/C2H6N4S/c3-1(4)6-2(5)7/h(H6,3,4,5,6,7) | |
Source | PubChem | |
URL | https://pubchem.ncbi.nlm.nih.gov | |
Description | Data deposited in or computed by PubChem | |
InChI Key |
OKGXJRGLYVRVNE-UHFFFAOYSA-N | |
Source | PubChem | |
URL | https://pubchem.ncbi.nlm.nih.gov | |
Description | Data deposited in or computed by PubChem | |
Canonical SMILES |
C(=NC(=S)N)(N)N | |
Source | PubChem | |
URL | https://pubchem.ncbi.nlm.nih.gov | |
Description | Data deposited in or computed by PubChem | |
Molecular Formula |
C2H6N4S | |
Source | PubChem | |
URL | https://pubchem.ncbi.nlm.nih.gov | |
Description | Data deposited in or computed by PubChem | |
DSSTOX Substance ID |
DTXSID50175378 | |
Record name | Guanylthiourea | |
Source | EPA DSSTox | |
URL | https://comptox.epa.gov/dashboard/DTXSID50175378 | |
Description | DSSTox provides a high quality public chemistry resource for supporting improved predictive toxicology. | |
Molecular Weight |
118.16 g/mol | |
Source | PubChem | |
URL | https://pubchem.ncbi.nlm.nih.gov | |
Description | Data deposited in or computed by PubChem | |
CAS No. |
2114-02-5 | |
Record name | Amidinothiourea | |
Source | CAS Common Chemistry | |
URL | https://commonchemistry.cas.org/detail?cas_rn=2114-02-5 | |
Description | CAS Common Chemistry is an open community resource for accessing chemical information. Nearly 500,000 chemical substances from CAS REGISTRY cover areas of community interest, including common and frequently regulated chemicals, and those relevant to high school and undergraduate chemistry classes. This chemical information, curated by our expert scientists, is provided in alignment with our mission as a division of the American Chemical Society. | |
Explanation | The data from CAS Common Chemistry is provided under a CC-BY-NC 4.0 license, unless otherwise stated. | |
Record name | Guanylthiourea | |
Source | ChemIDplus | |
URL | https://pubchem.ncbi.nlm.nih.gov/substance/?source=chemidplus&sourceid=0002114025 | |
Description | ChemIDplus is a free, web search system that provides access to the structure and nomenclature authority files used for the identification of chemical substances cited in National Library of Medicine (NLM) databases, including the TOXNET system. | |
Record name | 2114-02-5 | |
Source | DTP/NCI | |
URL | https://dtp.cancer.gov/dtpstandard/servlet/dwindex?searchtype=NSC&outputformat=html&searchlist=55743 | |
Description | The NCI Development Therapeutics Program (DTP) provides services and resources to the academic and private-sector research communities worldwide to facilitate the discovery and development of new cancer therapeutic agents. | |
Explanation | Unless otherwise indicated, all text within NCI products is free of copyright and may be reused without our permission. Credit the National Cancer Institute as the source. | |
Record name | Guanylthiourea | |
Source | EPA DSSTox | |
URL | https://comptox.epa.gov/dashboard/DTXSID50175378 | |
Description | DSSTox provides a high quality public chemistry resource for supporting improved predictive toxicology. | |
Record name | 1-amidino-2-thiourea | |
Source | European Chemicals Agency (ECHA) | |
URL | https://echa.europa.eu/substance-information/-/substanceinfo/100.016.644 | |
Description | The European Chemicals Agency (ECHA) is an agency of the European Union which is the driving force among regulatory authorities in implementing the EU's groundbreaking chemicals legislation for the benefit of human health and the environment as well as for innovation and competitiveness. | |
Explanation | Use of the information, documents and data from the ECHA website is subject to the terms and conditions of this Legal Notice, and subject to other binding limitations provided for under applicable law, the information, documents and data made available on the ECHA website may be reproduced, distributed and/or used, totally or in part, for non-commercial purposes provided that ECHA is acknowledged as the source: "Source: European Chemicals Agency, http://echa.europa.eu/". Such acknowledgement must be included in each copy of the material. ECHA permits and encourages organisations and individuals to create links to the ECHA website under the following cumulative conditions: Links can only be made to webpages that provide a link to the Legal Notice page. | |
Record name | GUANYLTHIOUREA | |
Source | FDA Global Substance Registration System (GSRS) | |
URL | https://gsrs.ncats.nih.gov/ginas/app/beta/substances/LF1NJY8ID8 | |
Description | The FDA Global Substance Registration System (GSRS) enables the efficient and accurate exchange of information on what substances are in regulated products. Instead of relying on names, which vary across regulatory domains, countries, and regions, the GSRS knowledge base makes it possible for substances to be defined by standardized, scientific descriptions. | |
Explanation | Unless otherwise noted, the contents of the FDA website (www.fda.gov), both text and graphics, are not copyrighted. They are in the public domain and may be republished, reprinted and otherwise used freely by anyone without the need to obtain permission from FDA. Credit to the U.S. Food and Drug Administration as the source is appreciated but not required. | |
Retrosynthesis Analysis
AI-Powered Synthesis Planning: Our tool employs the Template_relevance Pistachio, Template_relevance Bkms_metabolic, Template_relevance Pistachio_ringbreaker, Template_relevance Reaxys, Template_relevance Reaxys_biocatalysis model, leveraging a vast database of chemical reactions to predict feasible synthetic routes.
One-Step Synthesis Focus: Specifically designed for one-step synthesis, it provides concise and direct routes for your target compounds, streamlining the synthesis process.
Accurate Predictions: Utilizing the extensive PISTACHIO, BKMS_METABOLIC, PISTACHIO_RINGBREAKER, REAXYS, REAXYS_BIOCATALYSIS database, our tool offers high-accuracy predictions, reflecting the latest in chemical research and data.
Strategy Settings
Precursor scoring | Relevance Heuristic |
---|---|
Min. plausibility | 0.01 |
Model | Template_relevance |
Template Set | Pistachio/Bkms_metabolic/Pistachio_ringbreaker/Reaxys/Reaxys_biocatalysis |
Top-N result to add to graph | 6 |
Feasible Synthetic Routes
Q1: What is the molecular formula and weight of guanylthiourea?
A1: The molecular formula of guanylthiourea is C2H6N4S, and its molecular weight is 118.16 g/mol.
Q2: What are the key structural features of guanylthiourea?
A2: Guanylthiourea is characterized by the presence of both a thiourea group (-NH-CS-NH-) and a guanidine group (-NH-C(=NH)-NH2) within its structure. This unique combination contributes to its diverse reactivity and biological activity. []
Q3: Does guanylthiourea exhibit tautomerism?
A3: Yes, guanylthiourea displays tautomerism, primarily existing in two tautomeric forms: thione and thiol. These tautomers differ in the location of a proton and the position of a double bond. The thione form is generally more stable, but both contribute to the compound's reactivity. []
Q4: What spectroscopic techniques are used to characterize guanylthiourea?
A4: Various spectroscopic methods, including infrared (IR) and nuclear magnetic resonance (NMR) spectroscopy, are employed to analyze the structure of guanylthiourea. These techniques provide information about the functional groups present, their bonding environments, and the overall molecular structure. [, ]
Q5: How does guanylthiourea behave as a ligand in coordination chemistry?
A5: Guanylthiourea acts as a ligand, forming complexes with various metal ions, including silver(I) []. The sulfur atom in the thiourea group typically coordinates to the metal center. These complexes have been studied for their potential applications in areas like metal recovery from solutions. []
Q6: Can guanylthiourea be used as a nitrification inhibitor?
A6: Yes, research indicates that guanylthiourea can function as a nitrification inhibitor in soil [, , ]. It effectively inhibits the conversion of ammonium to nitrate, reducing nitrogen loss from fertilizers and potentially enhancing nitrogen use efficiency in agriculture. [, ]
Q7: What is the role of guanylthiourea in organic synthesis?
A7: Guanylthiourea serves as a versatile building block in organic synthesis. For example, it can be used to synthesize heterocyclic compounds like thiadiazoles and triazines. [, , ]
Q8: How does guanylthiourea contribute to the synthesis of biguanides?
A8: Guanylthiourea can be converted into biguanides, a class of compounds with various biological activities [, , ]. This conversion typically involves reactions with amines or amine derivatives.
Q9: Have computational chemistry methods been applied to study guanylthiourea?
A9: Yes, computational chemistry, particularly quantum chemical calculations, has been employed to investigate the electronic structure, tautomerism, and reactivity of guanylthiourea. [] These studies provide insights into the compound's behavior at the molecular level.
Q10: What is known about the structure-activity relationships (SAR) of guanylthiourea derivatives?
A10: Research on guanylthiourea derivatives, particularly in the context of antimalarial activity, has shed light on SAR [, ]. Modifications to the guanylthiourea core structure can significantly impact its biological activity, potency, and selectivity.
Q11: Does guanylthiourea exhibit any radioprotective properties?
A11: Studies have shown that guanylthiourea exhibits radioprotective effects in animal models [, , , ]. It is suggested that its mechanism of action might involve the protection of bone marrow cells from radiation damage.
Q12: What is the potential of guanylthiourea and its derivatives in medicinal chemistry?
A12: Guanylthiourea and its derivatives have been explored for their potential as antimalarial agents [, , ] and inhibitors of dihydrofolate reductase, an enzyme crucial for folate metabolism in parasites [].
Q13: Has guanylthiourea been investigated for its antityrosinase activity?
A13: Yes, research suggests that guanylthiourea exhibits antityrosinase activity [], indicating its potential as a skin-whitening agent by inhibiting the enzyme responsible for melanin production.
Q14: Is guanylthiourea stable under various environmental conditions?
A14: The stability of guanylthiourea can be influenced by factors like pH, temperature, and the presence of other chemicals. Studies have investigated its degradation in soil, providing insights into its environmental fate. []
Q15: What are the environmental implications of using guanylthiourea as a nitrification inhibitor?
A15: The use of guanylthiourea as a nitrification inhibitor can have both beneficial and potentially adverse effects on the environment. While it can reduce nitrate leaching and enhance nitrogen use efficiency, its degradation products and potential long-term impacts on soil ecosystems require further investigation. [, , , , ]
Descargo de responsabilidad e información sobre productos de investigación in vitro
Tenga en cuenta que todos los artículos e información de productos presentados en BenchChem están destinados únicamente con fines informativos. Los productos disponibles para la compra en BenchChem están diseñados específicamente para estudios in vitro, que se realizan fuera de organismos vivos. Los estudios in vitro, derivados del término latino "in vidrio", involucran experimentos realizados en entornos de laboratorio controlados utilizando células o tejidos. Es importante tener en cuenta que estos productos no se clasifican como medicamentos y no han recibido la aprobación de la FDA para la prevención, tratamiento o cura de ninguna condición médica, dolencia o enfermedad. Debemos enfatizar que cualquier forma de introducción corporal de estos productos en humanos o animales está estrictamente prohibida por ley. Es esencial adherirse a estas pautas para garantizar el cumplimiento de los estándares legales y éticos en la investigación y experimentación.