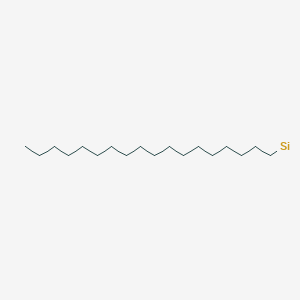
Octadecylsilane
Descripción general
Descripción
Tifacogin es una forma recombinante del inhibidor de la vía del factor tisular, una proteína que juega un papel crucial en la regulación de la coagulación sanguínea. Se ha investigado principalmente por su potencial uso terapéutico en afecciones como la sepsis grave y la neumonía adquirida en la comunidad .
Aplicaciones Científicas De Investigación
Tifacogin ha sido ampliamente estudiado por sus posibles aplicaciones terapéuticas:
Sepsis grave: Investigado por su capacidad para inhibir la coagulación y reducir la mortalidad en pacientes con sepsis grave
Neumonía adquirida en la comunidad: Estudiado como terapia adyuvante para mejorar los resultados en pacientes con neumonía grave.
Efectos antiinflamatorios: Explorado por sus propiedades antiinflamatorias en diversas afecciones inflamatorias.
Mecanismo De Acción
Tifacogin ejerce sus efectos inhibiendo la vía del factor tisular, un iniciador clave de la cascada de coagulación. Forma un complejo con el factor tisular y el factor VIIa, previniendo la activación del factor X y la posterior generación de trombina. Esta inhibición reduce la formación de coágulos de fibrina y modula las respuestas inflamatorias .
Compuestos similares:
Activador del plasminógeno tisular recombinante: Utilizado para la trombólisis en infarto agudo de miocardio y accidente cerebrovascular.
Antitrombina III: Un anticoagulante natural utilizado en la deficiencia hereditaria de antitrombina.
Comparación:
Singularidad: Tifacogin se dirige específicamente a la vía del factor tisular, mientras que el activador del plasminógeno tisular recombinante y la antitrombina III tienen efectos anticoagulantes más amplios.
El mecanismo específico de Tifacogin y sus posibles aplicaciones terapéuticas lo convierten en un compuesto único y valioso en el campo de la medicina.
Safety and Hazards
Direcciones Futuras
Análisis Bioquímico
Biochemical Properties
Octadecylsilane plays a significant role in biochemical reactions, particularly in the modification of silica surfaces. The compound interacts with silanol groups (Si–OH) on silica through chemical reactions, forming covalent bonds that enhance the hydrophobicity or hydrophilicity of the surface . This modification is crucial for applications such as incorporating silica particles into polymeric materials or increasing the hydrolytic stability of silica used in membranes . This compound also interacts with various biomolecules, including proteins, lipids, and nucleic acids, through hydrophobic interactions, which can influence the orientation and conformation of these biomolecules on modified surfaces .
Cellular Effects
This compound affects various types of cells and cellular processes. It influences cell function by modifying the surface properties of materials that cells interact with. For instance, the hydrophobic surface created by this compound can affect cell adhesion, proliferation, and differentiation . Additionally, the compound can impact cell signaling pathways, gene expression, and cellular metabolism by altering the microenvironment of cells . These effects are particularly relevant in the context of biosensors and biomaterials, where the interaction between cells and modified surfaces is critical for functionality.
Molecular Mechanism
The molecular mechanism of action of this compound involves its ability to form covalent bonds with silanol groups on silica surfaces, resulting in a modified surface with enhanced hydrophobicity . This modification can influence the binding interactions of biomolecules, such as proteins and lipids, with the surface. The hydrophobic interactions between this compound and biomolecules can lead to changes in the conformation and orientation of these biomolecules, affecting their biological activity . Additionally, this compound can modulate enzyme activity by altering the microenvironment and surface properties of the enzyme’s substrate .
Temporal Effects in Laboratory Settings
In laboratory settings, the effects of this compound can change over time due to its stability and degradation properties. The compound is generally stable under standard laboratory conditions, but its long-term effects on cellular function can vary depending on the specific application and environmental conditions . For instance, the hydrophobic surface created by this compound may undergo changes in wettability over time, which can influence the interaction between cells and the modified surface . Long-term studies have shown that the stability of this compound-modified surfaces can be maintained with proper storage and handling .
Dosage Effects in Animal Models
The effects of this compound vary with different dosages in animal models. At low doses, the compound is generally well-tolerated and can enhance the hydrophobicity of surfaces without causing significant adverse effects . At high doses, this compound may exhibit toxic effects, including irritation and inflammation, particularly in the respiratory system . Threshold effects have been observed, where the compound’s impact on cellular function and tissue integrity becomes more pronounced at higher concentrations .
Metabolic Pathways
This compound is involved in metabolic pathways related to its role as a surface modifier. The compound interacts with enzymes and cofactors that facilitate the modification of silica surfaces, including silanol groups and silicon alkoxides . These interactions can influence metabolic flux and metabolite levels by altering the surface properties of materials used in biochemical reactions . Additionally, this compound can affect the metabolism of cells by modifying the microenvironment and surface properties of cell culture substrates .
Transport and Distribution
This compound is transported and distributed within cells and tissues primarily through hydrophobic interactions with cellular membranes . The compound can interact with transporters and binding proteins that facilitate its movement within cells and tissues . Once inside the cell, this compound can accumulate in specific compartments, such as the cytoplasm and cell membrane, where it can exert its effects on cellular function .
Subcellular Localization
The subcellular localization of this compound is primarily in the cytoplasm and cell membrane . The compound’s hydrophobic nature allows it to integrate into lipid bilayers and interact with membrane proteins . This localization can influence the activity and function of this compound by affecting its interactions with other biomolecules and cellular structures . Additionally, targeting signals and post-translational modifications may direct this compound to specific compartments or organelles within the cell .
Métodos De Preparación
Rutas sintéticas y condiciones de reacción: Tifacogin se produce utilizando tecnología de ADN recombinante. El gen que codifica el inhibidor de la vía del factor tisular se inserta en un vector de expresión adecuado, que luego se introduce en una línea celular huésped, típicamente células de ovario de hámster chino. Las células se cultivan en condiciones controladas para expresar la proteína, que posteriormente se purifica mediante técnicas cromatográficas .
Métodos de producción industrial: La producción industrial de tifacogin implica la fermentación a gran escala de las células modificadas genéticamente. El proceso incluye varios pasos:
Cultivo celular: Las células huésped se cultivan en biorreactores con un medio rico en nutrientes.
Expresión de proteínas: Inducción de la expresión de proteínas a través de condiciones específicas.
Cosecha: Las células se cosechan y se extrae la proteína.
Purificación: La proteína se somete a múltiples pasos de purificación, incluida la cromatografía de afinidad y la cromatografía de intercambio iónico, para lograr una alta pureza
Análisis De Reacciones Químicas
Tipos de reacciones: Tifacogin, al ser una proteína, principalmente se somete a reacciones bioquímicas en lugar de reacciones químicas tradicionales. Estas incluyen:
Proteólisis: Descomposición de la proteína por proteasas.
Glucosilación: Adición de grupos de carbohidratos a la proteína.
Oxidación: Modificaciones oxidativas de residuos de aminoácidos
Reactivos y condiciones comunes:
Proteólisis: Enzimas como la tripsina o la pepsina.
Glucosilación: Enzimas como las glucosiltransferasas.
Oxidación: Especies reactivas de oxígeno o agentes oxidantes específicos
Principales productos formados:
Proteólisis: Fragmentos peptídicos más pequeños.
Glucosilación: Glucoproteínas con propiedades funcionales alteradas.
Oxidación: Formas de proteína oxidada con posibles cambios en la actividad
Comparación Con Compuestos Similares
Recombinant Tissue Plasminogen Activator: Used for thrombolysis in acute myocardial infarction and stroke.
Antithrombin III: A natural anticoagulant used in hereditary antithrombin deficiency.
Comparison:
Uniqueness: Tifacogin specifically targets the tissue factor pathway, whereas recombinant tissue plasminogen activator and antithrombin III have broader anticoagulant effects.
Tifacogin’s targeted mechanism and potential therapeutic applications make it a unique and valuable compound in the field of medicine.
Propiedades
InChI |
InChI=1S/C18H37Si/c1-2-3-4-5-6-7-8-9-10-11-12-13-14-15-16-17-18-19/h2-18H2,1H3 | |
---|---|---|
Source | PubChem | |
URL | https://pubchem.ncbi.nlm.nih.gov | |
Description | Data deposited in or computed by PubChem | |
InChI Key |
FUESFIVIIFEDFI-UHFFFAOYSA-N | |
Source | PubChem | |
URL | https://pubchem.ncbi.nlm.nih.gov | |
Description | Data deposited in or computed by PubChem | |
Canonical SMILES |
CCCCCCCCCCCCCCCCCC[Si] | |
Source | PubChem | |
URL | https://pubchem.ncbi.nlm.nih.gov | |
Description | Data deposited in or computed by PubChem | |
Molecular Formula |
C18H37Si | |
Source | PubChem | |
URL | https://pubchem.ncbi.nlm.nih.gov | |
Description | Data deposited in or computed by PubChem | |
DSSTOX Substance ID |
DTXSID20885058 | |
Record name | Silane, octadecyl- | |
Source | EPA DSSTox | |
URL | https://comptox.epa.gov/dashboard/DTXSID20885058 | |
Description | DSSTox provides a high quality public chemistry resource for supporting improved predictive toxicology. | |
Molecular Weight |
281.6 g/mol | |
Source | PubChem | |
URL | https://pubchem.ncbi.nlm.nih.gov | |
Description | Data deposited in or computed by PubChem | |
CAS No. |
18623-11-5 | |
Record name | Octadecylsilane | |
Source | ChemIDplus | |
URL | https://pubchem.ncbi.nlm.nih.gov/substance/?source=chemidplus&sourceid=0018623115 | |
Description | ChemIDplus is a free, web search system that provides access to the structure and nomenclature authority files used for the identification of chemical substances cited in National Library of Medicine (NLM) databases, including the TOXNET system. | |
Record name | Silane, octadecyl- | |
Source | EPA Chemicals under the TSCA | |
URL | https://www.epa.gov/chemicals-under-tsca | |
Description | EPA Chemicals under the Toxic Substances Control Act (TSCA) collection contains information on chemicals and their regulations under TSCA, including non-confidential content from the TSCA Chemical Substance Inventory and Chemical Data Reporting. | |
Record name | Silane, octadecyl- | |
Source | EPA DSSTox | |
URL | https://comptox.epa.gov/dashboard/DTXSID20885058 | |
Description | DSSTox provides a high quality public chemistry resource for supporting improved predictive toxicology. | |
Record name | Octadecylsilane | |
Source | European Chemicals Agency (ECHA) | |
URL | https://echa.europa.eu/substance-information/-/substanceinfo/100.038.579 | |
Description | The European Chemicals Agency (ECHA) is an agency of the European Union which is the driving force among regulatory authorities in implementing the EU's groundbreaking chemicals legislation for the benefit of human health and the environment as well as for innovation and competitiveness. | |
Explanation | Use of the information, documents and data from the ECHA website is subject to the terms and conditions of this Legal Notice, and subject to other binding limitations provided for under applicable law, the information, documents and data made available on the ECHA website may be reproduced, distributed and/or used, totally or in part, for non-commercial purposes provided that ECHA is acknowledged as the source: "Source: European Chemicals Agency, http://echa.europa.eu/". Such acknowledgement must be included in each copy of the material. ECHA permits and encourages organisations and individuals to create links to the ECHA website under the following cumulative conditions: Links can only be made to webpages that provide a link to the Legal Notice page. | |
Record name | OCTADECYLSILANE | |
Source | FDA Global Substance Registration System (GSRS) | |
URL | https://gsrs.ncats.nih.gov/ginas/app/beta/substances/62PU8XK4BV | |
Description | The FDA Global Substance Registration System (GSRS) enables the efficient and accurate exchange of information on what substances are in regulated products. Instead of relying on names, which vary across regulatory domains, countries, and regions, the GSRS knowledge base makes it possible for substances to be defined by standardized, scientific descriptions. | |
Explanation | Unless otherwise noted, the contents of the FDA website (www.fda.gov), both text and graphics, are not copyrighted. They are in the public domain and may be republished, reprinted and otherwise used freely by anyone without the need to obtain permission from FDA. Credit to the U.S. Food and Drug Administration as the source is appreciated but not required. | |
Retrosynthesis Analysis
AI-Powered Synthesis Planning: Our tool employs the Template_relevance Pistachio, Template_relevance Bkms_metabolic, Template_relevance Pistachio_ringbreaker, Template_relevance Reaxys, Template_relevance Reaxys_biocatalysis model, leveraging a vast database of chemical reactions to predict feasible synthetic routes.
One-Step Synthesis Focus: Specifically designed for one-step synthesis, it provides concise and direct routes for your target compounds, streamlining the synthesis process.
Accurate Predictions: Utilizing the extensive PISTACHIO, BKMS_METABOLIC, PISTACHIO_RINGBREAKER, REAXYS, REAXYS_BIOCATALYSIS database, our tool offers high-accuracy predictions, reflecting the latest in chemical research and data.
Strategy Settings
Precursor scoring | Relevance Heuristic |
---|---|
Min. plausibility | 0.01 |
Model | Template_relevance |
Template Set | Pistachio/Bkms_metabolic/Pistachio_ringbreaker/Reaxys/Reaxys_biocatalysis |
Top-N result to add to graph | 6 |
Feasible Synthetic Routes
Q1: What is the molecular formula and weight of octadecylsilane?
A1: While the provided research articles don't explicitly state the molecular formula and weight of this compound, it's essential to clarify that the term "this compound" generally refers to a family of compounds with an 18-carbon alkyl chain (octadecyl) bonded to a silicon atom. The specific formula and weight depend on the substituents attached to the silicon atom. For example, octadecyltrichlorosilane, a common precursor for ODS stationary phases, has the formula CH3(CH2)17SiCl3 and a molecular weight of 387.8 g/mol.
Q2: How can Raman spectroscopy be used to characterize this compound stationary phases?
A2: Raman spectroscopy provides insights into the alkyl chain conformation of ODS stationary phases. Spectral data, particularly in the ν(C-C) and ν(C-H) regions, help assess the conformational order and its dependence on factors like temperature, surface coverage, and solvent environment [, , , , , , ]. This information is crucial for understanding the separation mechanisms and selectivity of ODS-based chromatographic columns.
Q3: How stable are this compound stationary phases under different conditions?
A3: The stability of ODS stationary phases depends on factors like the type of silica substrate, bonding density, and eluent composition. Research indicates that multifunctionally bonded ODS phases generally exhibit higher resistance to ligand stripping, especially in high pH eluents, compared to monofunctionally modified phases [].
Q4: What role does the silica substrate play in the stability of this compound stationary phases?
A4: The rigidity of the silica substrate influences the stability of the bonded phase, especially for monofunctionally modified ODS []. Using multifunctional silanes for modification can mitigate the influence of substrate characteristics on the stability compared to monofunctional ODS.
Q5: How does this compound function as a stationary phase in high-performance liquid chromatography (HPLC)?
A5: ODS is widely employed as a stationary phase in reversed-phase HPLC due to its hydrophobic nature. Analytes in the mobile phase interact with the nonpolar ODS chains through hydrophobic interactions, leading to their separation based on their relative hydrophobicity [, , , , , , , ].
Q6: What are the advantages of using this compound functionalized graphene oxide/silica composite as a stationary phase in HPLC?
A6: Incorporating graphene oxide (GO) into the ODS/silica stationary phase introduces π-π interactions between the analyte and the stationary phase, in addition to the typical hydrophobic interactions observed with traditional C18 columns. This additional interaction mechanism can enhance the separation of certain analytes, particularly those with aromatic rings [].
Q7: How do different mobile phase solvents affect the performance of this compound stationary phases?
A7: The type of solvent used in the mobile phase significantly influences the conformational order and solvation of the ODS stationary phase [, ]. Polar, hydrogen-bonding solvents tend to induce rotational ordering in high-density ODS phases, while hydrophobic solvents lead to greater disorder. Understanding these interactions is crucial for optimizing separation efficiency and selectivity in HPLC.
Q8: Can this compound be used for the separation of specific compounds or classes of compounds?
A8: Yes, ODS stationary phases are versatile and used for separating a wide range of compounds, including alkylbenzenes, polycyclic aromatic hydrocarbons, amines, phenolic compounds, triglycerides, fat-soluble vitamins, pesticides, and pharmaceuticals [, , , , , , , ]. The choice of mobile phase composition, temperature, and flow rate can be adjusted to optimize the separation of specific analytes.
Q9: How does the surface coverage of this compound on the silica support affect its chromatographic performance?
A9: Higher surface coverage of ODS generally leads to increased alkyl chain order and enhances shape selectivity in chromatographic separations [, , , ]. High-density ODS phases are particularly useful for separating structurally similar compounds that differ slightly in their shape or size.
Q10: What analytical techniques are commonly employed to quantify this compound in various matrices?
A10: While the provided research mainly focuses on the characterization and application of ODS-modified materials, techniques like elemental analysis and solid-state NMR can be used to quantify ODS loading on silica supports []. In the context of chromatographic applications, various detectors are employed depending on the analyte being analyzed, including UV detectors, mass spectrometers, and fluorescence detectors [, , , , ].
Q11: How does this compound contribute to the creation of optically anisotropic thin films?
A11: ODS, when combined with perylenesilane at liquid-liquid interfaces, can be used to create optically anisotropic 2D silica films []. These films exhibit varying wetting behaviors depending on the ODS concentration, highlighting the potential for developing sensitive and smart coatings.
Q12: What is the role of this compound in controlling the growth of organic semiconductors in thin-film applications?
A12: The density of the terminal methyl groups in ODS monolayers plays a crucial role in directing the growth of organic semiconductors like pentacene []. Crystalline, densely packed ODS layers promote two-dimensional pentacene growth, favorable for charge transport in organic thin-film transistors. In contrast, amorphous ODS layers lead to less desirable three-dimensional growth.
Q13: How is this compound used in the development of superhydrophobic surfaces?
A13: Polymerized n-octadecylsilane with micronano hierarchical structures created on quartz crystal microbalance (QCM) surfaces exhibits superhydrophobic properties []. These surfaces show promise as sensing materials for detecting volatile organic compounds, demonstrating the potential of ODS in sensor technology.
Descargo de responsabilidad e información sobre productos de investigación in vitro
Tenga en cuenta que todos los artículos e información de productos presentados en BenchChem están destinados únicamente con fines informativos. Los productos disponibles para la compra en BenchChem están diseñados específicamente para estudios in vitro, que se realizan fuera de organismos vivos. Los estudios in vitro, derivados del término latino "in vidrio", involucran experimentos realizados en entornos de laboratorio controlados utilizando células o tejidos. Es importante tener en cuenta que estos productos no se clasifican como medicamentos y no han recibido la aprobación de la FDA para la prevención, tratamiento o cura de ninguna condición médica, dolencia o enfermedad. Debemos enfatizar que cualquier forma de introducción corporal de estos productos en humanos o animales está estrictamente prohibida por ley. Es esencial adherirse a estas pautas para garantizar el cumplimiento de los estándares legales y éticos en la investigación y experimentación.