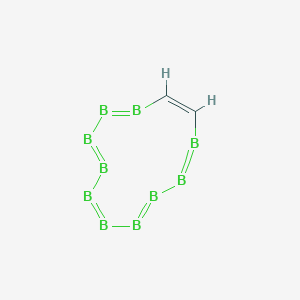
o-Carborane
- Haga clic en CONSULTA RÁPIDA para recibir una cotización de nuestro equipo de expertos.
- Con productos de calidad a un precio COMPETITIVO, puede centrarse más en su investigación.
Descripción general
Descripción
o-Carborane (1,2-dicarba-closo-dodecaborane, C₂B₁₀H₁₂) is an icosahedral boron cluster with two adjacent carbon atoms and ten boron atoms arranged in a three-dimensional aromatic structure. Its unique electronic properties arise from delocalized 3-center-2-electron bonds, conferring σ-aromaticity and strong electron-withdrawing characteristics . The C–C bond in the carborane cage (1.629–1.743 Å) is highly sensitive to substituents, with elongation observed upon aryl or metal complexation, altering electronic and photophysical behaviors .
This compound is a cornerstone in materials science and biomedicine. Its applications span boron neutron capture therapy (BNCT) due to high boron content , aggregation-induced emission (AIE) materials , and stimuli-responsive luminochromic systems . The electron-deficient cage facilitates intramolecular charge transfer (ICT) when conjugated with aromatic groups, enabling tunable optoelectronic properties .
Métodos De Preparación
Traditional Synthesis from Decaborane and Acetylenes
The classical route to o-carborane involves the direct reaction of decaborane (B₁₀H₁₄) with acetylene derivatives. This method, first reported in the 1960s, remains foundational despite its limitations in yield and reproducibility .
Reaction Mechanism and Conditions
Decaborane reacts with acetylenes in inert solvents (e.g., benzene, toluene) at 80–100°C under nitrogen . The process initiates with the formation of a B₁₀H₁₂·L₂ adduct (L = Lewis base like Et₂S), which reacts with acetylene to replace two boron vertices with carbon atoms . Terminal alkynes (RC≡CH) yield 1-substituted o-carboranes, while acetylene gas produces the parent cluster . Symmetrical internal alkynes (RC≡CR') are less reactive due to steric hindrance, though unsymmetrical variants show moderate activity .
Limitations of Conventional Methods
Traditional synthesis suffers from low yields (40–60%), prolonged reaction times (24–48 hours), and sensitivity to moisture . For example, the reaction of decaborane with phenylacetylene in toluene yields only 58% this compound after 24 hours . Additionally, purification often requires multiple recrystallizations, complicating large-scale production.
Modern Innovations in this compound Synthesis
Recent advancements focus on enhancing efficiency, reducing reaction times, and improving selectivity. Key strategies include microwave-assisted synthesis, ionic liquid media, and metal catalysis.
Microwave-Activated Reactions
Microwave (MW) irradiation accelerates this compound formation by enabling rapid, uniform heating. In acetonitrile at 120°C, MW irradiation reduces reaction times to 1–20 minutes, achieving yields of 63–91% for terminal alkynes (Table 1) . For instance, 1-octyne reacts with decaborane under MW to yield 85% this compound in 1 minute, compared to 23 hours via conventional heating . This method also minimizes side reactions, such as deboronation, which are prevalent in prolonged thermal exposure .
Table 1. Yields of this compound Derivatives Under Microwave Irradiation
Alkyne | Solvent/Medium | Time (min) | Yield (%) |
---|---|---|---|
1-Octyne | [bmim]Cl | 1 | 85 |
Phenylacetylene | Acetonitrile | 20 | 65 |
Propargyl ether | [bmim]Cl | 1 | 91 |
Ionic Liquids as Reaction Media
Ionic liquids (e.g., 1-butyl-3-methylimidazolium chloride, [bmim]Cl) promote decaborane ionization to B₁₀H₁₃⁻, enhancing reactivity with acetylenes . Reactions in [bmim]Cl at 120°C achieve yields exceeding 85% within 1–7 minutes, outperforming traditional solvents . The ionic liquid also acts as a phase-transfer catalyst, simplifying product isolation via extraction .
Silver-Catalyzed Synthesis
Silver ions (Ag⁺) catalyze this compound formation by stabilizing reactive intermediates. In acetonitrile with AgNO₃, decaborane and phenylacetylene react at 80°C to yield 78% this compound in 2 hours . The catalytic cycle involves Ag⁺ coordinating to the alkyne, facilitating boron-carbon bond formation . This method is particularly effective for electron-deficient acetylenes, which are less reactive under traditional conditions.
Functionalization and Derivative Synthesis
While this article focuses on core this compound preparation, functionalization methods are critical for tailoring properties. Key approaches include iodination, arylation, and cross-coupling reactions.
Regioselective Iodination
Iodination of 1-substituted o-carboranes with ICl yields 1,12-diiodo derivatives, which serve as precursors for further functionalization . For example, 1-phenyl-o-carborane reacts with ICl to form 1-iodo-12-phenyl-o-carborane in 32% yield after chromatographic separation . These iodinated derivatives enable Suzuki-Miyaura and Negishi couplings for attaching aromatic or aliphatic groups .
Transition-Metal-Free Arylation
A base-mediated intramolecular C-arylation method produces arene-fused o-carboranes without metals. Treatment of 1-(8-bromo-1-naphthyl)-o-carborane with KOtBu in THF yields naphthyl-fused this compound (ΦPL = 0.79), a fluoranthene analogue with enhanced fluorescence . This approach avoids metal contamination, making it suitable for optoelectronic applications .
Comparative Analysis of Synthesis Methods
The table below summarizes key metrics for major this compound preparation techniques:
Table 2. Efficiency Metrics for this compound Synthesis
Method | Time | Yield (%) | Selectivity | Scalability |
---|---|---|---|---|
Traditional (B₁₀H₁₄) | 24–48 h | 40–60 | Moderate | Low |
Microwave/IL | 1–20 min | 63–91 | High | High |
AgNO₃ Catalysis | 2–6 h | 70–78 | High | Moderate |
Microwave-assisted synthesis in ionic liquids emerges as the most efficient, combining rapid kinetics with high yields . However, silver catalysis offers better control over electron-poor substrates .
Análisis De Reacciones Químicas
DMT-dG(ib) Phosphoramidite undergoes several types of reactions:
Deprotection: The DMT group is removed using acidic conditions, typically with trichloroacetic acid in dichloromethane.
Cleavage and Deprotection: The final oligonucleotide is cleaved from the solid support and deprotected using concentrated ammonia solution.
Common reagents include iodine, trichloroacetic acid, and concentrated ammonia solution. The major products formed are the desired oligonucleotides with high purity and yield .
Aplicaciones Científicas De Investigación
Materials Science
- Porous Materials : Recent studies have demonstrated the synthesis of o-carborane-based π-conjugated macrocycles that exhibit excellent photophysical properties and micropore characteristics. These macrocycles have shown potential as sensors for nitroaromatics due to their fluorescence quenching behavior when exposed to specific compounds like 1,4-dinitrobenzene .
- Metal-Organic Frameworks (MOFs) : Carborane-based MOFs have been developed for applications in gas storage, separation, and catalysis under harsh conditions. Functionalized o-carboranes can endow these frameworks with switchable hydrophobicity and superhydrophilicity, enhancing their utility in various environmental applications .
Application Area | Material Type | Key Properties |
---|---|---|
Gas Storage | Carborane-based MOFs | High porosity, switchable properties |
Sensors | π-Conjugated Macrocycles | Fluorescence quenching |
Medicine
- Boron Neutron Capture Therapy (BNCT) : this compound derivatives are being explored as potential agents in BNCT due to their ability to selectively target cancer cells. Studies involving chromium(0) tricarbonyl complexes of this compound have shown promising biological activity, suggesting their viability as therapeutic agents .
- Drug Delivery Systems : Carborane-based covalent organic frameworks (COFs) have been utilized to encapsulate drugs, enhancing stability and solubility for improved pharmacokinetics. These systems can potentially overcome limitations associated with conventional small-molecule drugs in cancer therapy .
Application Area | Compound Type | Key Benefits |
---|---|---|
Cancer Therapy | BNCT Agents | Selective targeting |
Drug Delivery | Carborane-loaded COFs | Enhanced stability and solubility |
Catalysis
- Functionalization : The controlled functionalization of this compound via transition metal catalysis has opened new avenues for creating functional building blocks in organometallic chemistry. This includes vertex-specific B–H activation that allows for selective modifications of the carborane structure, making it suitable for various catalytic applications .
- Environmental Applications : Research has indicated that o-carboranes can be employed in environmental remediation processes, such as the degradation of organic pollutants through advanced oxidation processes .
Synthesis of π-Conjugated Macrocycles
A study focused on synthesizing two this compound-based π-conjugated macrocycles revealed their unique porous structures and potential application as sensors for nitroaromatic compounds. The macrocycles exhibited aggregation-induced emission properties, making them suitable candidates for optical sensing technologies .
Development of Carborane-Based MOFs
Research on carborane-based MOFs highlighted their dual functionality—acting as both adsorbents and catalysts under varying environmental conditions. The reversible switching between hydrophobic and superhydrophilic states was demonstrated through experimental cycles, showcasing their adaptability for practical applications in gas separation technologies .
Mecanismo De Acción
DMT-dG(ib) Fosforamidita funciona incorporándose a la cadena de ADN en crecimiento durante la síntesis de oligonucleótidos. El grupo isobutirilo protege la base guanina, previniendo reacciones secundarias no deseadas. El grupo DMT protege el grupo hidroxilo 5’, permitiendo la desprotección y el acoplamiento selectivos durante la síntesis . El grupo fosforamidita facilita la formación de triésteres de fosfito, que posteriormente se oxidan a triésteres de fosfato estables .
Comparación Con Compuestos Similares
Structural and Electronic Differences Among Carborane Isomers
o-Carborane is compared with its isomers m-carborane (1,7-C₂B₁₀H₁₂) and p-carborane (1,12-C₂B₁₀H₁₂):
- Reactivity : this compound undergoes electrophilic substitution at boron or carbon atoms, guided by HSAB theory. Its C–H bonds are more acidic (pKa ~23) than those in m- or p-carboranes, enabling deprotonation and nucleophilic substitution .
- Isomerization : this compound irreversibly rearranges to m-carborane under thermal stress, accelerated by bulky substituents (e.g., 1,2-diphenyl-o-carborane isomerizes 10× faster than unsubstituted this compound) .
2.2.1. Benzene Analogs
This compound is termed an "inorganic benzene" due to aromaticity but differs critically:
Property | This compound | Benzene |
---|---|---|
Aromaticity Type | σ-Aromatic | π-Aromatic |
Electron Effect | Electron-withdrawing | Electron-donating |
Bond Polarization | High (C–C bond polarized) | Low |
Applications | BNCT, AIE materials | Traditional organic synthesis |
- ICT vs. π-π* Transitions : this compound-aryl conjugates exhibit redshifted emission (e.g., 552 nm in toluene) via ICT, unlike benzene’s π-π* transitions .
2.2.2. Bis(phenyl)-o-carborane vs. Triphenyl-o-carborane
Substitution patterns dictate reactivity and photophysics:
- Stoichiometric Reactivity: 1,2-Diphenyl-o-carborane reacts with Cr(CO)₆ to form mono-/bis-chromium complexes (yields: 45–60%), whereas triphenyl derivatives show lower reactivity .
Luminescent Properties vs. m-Carborane Derivatives
This compound’s AIE and stimuli-responsive emission contrast with m-carborane’s behavior:
- Excimer Formation : this compound derivatives exhibit solid-state excimer emission (e.g., 482 nm in PMMA films), absent in m-carborane systems .
Actividad Biológica
o-Carborane, a member of the carborane family, is a unique compound composed of boron and carbon atoms arranged in an icosahedral structure. This compound has garnered attention in various fields, particularly in medicinal chemistry, due to its intriguing biological properties and potential applications in targeted therapies. Its structural characteristics contribute to its stability and ability to interact with biological systems, making it a candidate for drug design and therapeutic applications.
Structural Characteristics
This compound has a three-dimensional aromatic character which enhances its interactions with biological targets. The unique arrangement of boron atoms allows for increased hydrophobic interactions, improving the bioavailability and stability of compounds that incorporate this compound structures. This property is particularly relevant in the design of inhibitors targeting enzymes overexpressed in certain cancers.
1. Inhibition of Carbonic Anhydrase
A significant study demonstrated that carborane-containing compounds can inhibit Carbonic Anhydrase IX (CAIX), an enzyme overexpressed in several cancers, including mesothelioma and breast cancer. The sulfonamido-functionalized carborane (CA-SF) was designed to act as both a CAIX inhibitor and a boron delivery agent for Boron Neutron Capture Therapy (BNCT). In vitro studies showed that CA-SF effectively hampered tumor growth by delivering therapeutic doses of boron to cancer cells while simultaneously inhibiting CAIX activity .
2. Boron Neutron Capture Therapy (BNCT)
This compound's role in BNCT is noteworthy. BNCT is a targeted cancer therapy that utilizes boron-containing compounds to selectively kill cancer cells upon neutron irradiation. Studies have indicated that this compound derivatives can serve as effective boron delivery agents, leading to enhanced therapeutic outcomes in cancers such as glioblastoma and head and neck tumors. The mechanism involves the capture of thermal neutrons by the non-radioactive boron isotope, resulting in localized cell damage .
3. Cytotoxicity Studies
Recent research involving 1,2-diphenyl-o-carborane and its chromium derivatives revealed promising cytotoxic effects against various cancer cell lines (e.g., B16 and CT26). The study evaluated how structural modifications influenced biological activity, finding that certain chromium-substituted o-carboranes exhibited higher cytotoxicity than traditional boron-containing agents like p-boronophenylalanine (BPA) .
Case Studies
Study | Compound | Target | Outcome |
---|---|---|---|
Nature (2020) | CA-SF | CAIX | Significant inhibition of tumor growth in mesothelioma models with enhanced boron delivery for BNCT |
MDPI (2023) | 1,2-Diphenyl-o-carborane | B16/CT26 cancer cells | Higher cytotoxicity compared to BPA; potential for BNCT applications |
RSC Advances (2023) | Functionalized o-carboranes | Various cancer cell lines | Demonstrated selective binding and inhibition of cancer cell growth |
Mechanistic Insights
The biological activity of o-carboranes can be attributed to several mechanisms:
- Enzyme Inhibition : The structural features of o-carboranes allow them to bind effectively to active sites on enzymes like CAIX, thereby inhibiting their function.
- Selective Targeting : The ability of o-carboranes to accumulate preferentially in tumor tissues enhances their efficacy as therapeutic agents.
- Synergistic Effects : When combined with neutron irradiation in BNCT, the cytotoxic effects are amplified due to the localized damage caused by the decay of boron isotopes.
Q & A
Basic Research Questions
Q. What are the key considerations when designing experiments to synthesize o-carborane derivatives with specific functional groups?
- Methodological Answer : Prioritize selecting boron-rich precursors (e.g., decaborane) and optimize reaction conditions (e.g., temperature, solvent polarity) to control substitution patterns. Purification via column chromatography or recrystallization is critical, followed by characterization using 11B NMR (to confirm boron cage integrity) and FT-IR (B–H stretching at ~2589 cm−1) . For reproducibility, document stoichiometric ratios and reaction times, adhering to guidelines for reporting experimental details .
Q. How should researchers address inconsistencies in spectroscopic data during this compound characterization?
- Methodological Answer : Cross-validate 1H and 13C NMR data with X-ray crystallography to resolve ambiguities in cage geometry. For example, discrepancies in 11B NMR signals may arise from dynamic cage distortions; use variable-temperature NMR to probe rotational dynamics .
Q. What statistical tools are recommended for analyzing crystallographic data of this compound complexes?
- Methodological Answer : Employ software like Mercury or OLEX2 for structural refinement. Use density functional theory (DFT) calculations (e.g., PBE0/6-31G(d)) to correlate experimental bond angles with computational models, particularly for non-covalent interactions (e.g., B–H⋯π) .
Advanced Research Questions
Q. What strategies can reconcile contradictions between computational predictions and experimental observations in this compound rotational dynamics?
- Methodological Answer : Perform potential energy surface scans (e.g., dihedral angles from −180° to 180°) to model rotational barriers. Compare DFT-derived energy profiles (e.g., relative S0-state energies) with X-ray data (e.g., J = 82.7–87.1° vs. computed −88°) to identify solvent or lattice effects . Use molecular dynamics simulations to account for environmental perturbations .
Q. How can researchers optimize this compound-based dendritic glycoconjugates for targeted boron neutron capture therapy (BNCT)?
- Methodological Answer : Incorporate multiple this compound clusters (e.g., nine glucose moieties per dendrimer) to enhance boron content. Validate cellular uptake via fluorescence tagging and assess cytotoxicity using MTT assays. Monitor B–H activation via 1H NMR (δ 85–86 ppm) and FT-IR (B–H stretching) to ensure stability in physiological conditions .
Q. What mechanistic insights guide the design of this compound oxidation reactions under mild conditions?
- Methodological Answer : Probe electrophilic attack pathways using HNO3, where B(9)–H acts as a nucleophilic site. Track H(-I) → H(I) transitions via isotopic labeling (2H/3H) and kinetic isotope effects. Validate intermediates using LC-MS and computational studies (e.g., NBO analysis) .
Q. How do chalcogen bonding (ChB) interactions influence the supramolecular assembly of this compound derivatives?
- Methodological Answer : Design this compound analogs with selenium or tellurium substituents. Characterize ChB-driven crystal packing via single-crystal XRD (e.g., Se⋯B distances < 3.5 Å) and Hirshfeld surface analysis. Compare with hydrogen-bonded networks to assess stability .
Q. Data Analysis and Contradiction Resolution
Q. What protocols mitigate discrepancies in quantifying boron content in this compound-based materials?
- Methodological Answer : Use ICP-MS for absolute boron quantification, calibrated against certified standards. Cross-check with combustion analysis (e.g., elemental CHN data) to detect impurities. For conflicting results, repeat measurements under inert atmospheres to prevent oxidation .
Q. How can researchers validate the purity of novel this compound compounds when commercial standards are unavailable?
Propiedades
Número CAS |
16872-09-6 |
---|---|
Fórmula molecular |
C2H2B10 |
Peso molecular |
134.2 g/mol |
Nombre IUPAC |
1,2,3,4,5,6,7,8,9,10-decaboracyclododeca-1,3,5,7,9,11-hexaene |
InChI |
InChI=1S/C2H2B10/c1-2-4-6-8-10-12-11-9-7-5-3-1/h1-2H |
Clave InChI |
GJLPUBMCTFOXHD-UHFFFAOYSA-N |
SMILES |
B1=BB=BB=BC=CB=BB=B1 |
SMILES isomérico |
B1=BB=BB=B/C=C\B=BB=B1 |
SMILES canónico |
B1=BB=BB=BC=CB=BB=B1 |
Key on ui other cas no. |
16872-09-6 |
Descripción física |
White powder, insoluble in water; [MSDSonline] |
Pictogramas |
Flammable; Irritant |
Origen del producto |
United States |
Descargo de responsabilidad e información sobre productos de investigación in vitro
Tenga en cuenta que todos los artículos e información de productos presentados en BenchChem están destinados únicamente con fines informativos. Los productos disponibles para la compra en BenchChem están diseñados específicamente para estudios in vitro, que se realizan fuera de organismos vivos. Los estudios in vitro, derivados del término latino "in vidrio", involucran experimentos realizados en entornos de laboratorio controlados utilizando células o tejidos. Es importante tener en cuenta que estos productos no se clasifican como medicamentos y no han recibido la aprobación de la FDA para la prevención, tratamiento o cura de ninguna condición médica, dolencia o enfermedad. Debemos enfatizar que cualquier forma de introducción corporal de estos productos en humanos o animales está estrictamente prohibida por ley. Es esencial adherirse a estas pautas para garantizar el cumplimiento de los estándares legales y éticos en la investigación y experimentación.