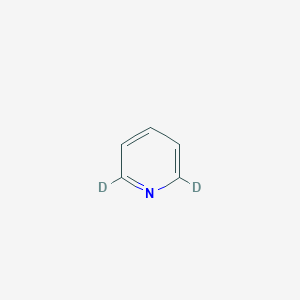
Piridina-2,6-d2
Descripción general
Descripción
Pyridine-2,6-d2 is a deuterated derivative of pyridine, where two hydrogen atoms at the 2 and 6 positions of the pyridine ring are replaced by deuterium atoms. This compound is of significant interest in various fields of scientific research due to its unique isotopic properties, which can be utilized in spectroscopic studies and kinetic isotope effect investigations.
Aplicaciones Científicas De Investigación
Pyridine-2,6-d2 has a wide range of applications in scientific research:
Chemistry: Used as a solvent and reagent in organic synthesis. Its deuterated form is valuable in nuclear magnetic resonance (NMR) spectroscopy for studying reaction mechanisms and molecular structures.
Biology: Employed in isotope labeling studies to trace metabolic pathways and enzyme mechanisms.
Medicine: Utilized in the development of deuterated drugs, which can exhibit improved pharmacokinetic properties.
Industry: Applied in the production of specialty chemicals and materials with enhanced properties due to the presence of deuterium.
Mecanismo De Acción
Target of Action
It has been used in the synthesis of new heterocyclic polyurethane materials . It’s also been reported to have significant roles in coordination chemistry, stabilization of reactive species, synthetic modeling of some metalloenzyme active sites, catalytic organic transformations, and sensing .
Mode of Action
It has been reported that the inclusion of the pyridine derivative into polyurethane urea chain structure had been successful . The obtained polyurethane matrix structure was characterized through fewer specific intermolecular hydrogen bonds between the pyridine units, urethane, and amide groups .
Biochemical Pathways
It has been involved in the synthesis of new heterocyclic polyurethane materials . It’s also been reported to have roles in coordination chemistry, stabilization of reactive species, synthetic modeling of some metalloenzyme active sites, catalytic organic transformations, and sensing .
Pharmacokinetics
A study reported that a polymer-interferon alpha conjugate synthesized using pyridine-2,6-dicarboxaldehyde showed significantly improved pharmacokinetics .
Result of Action
It has been reported that the introduction of 2,6-pyridinedicarboxamide into the main chains of linear and crosslinked polyurethanes resulted in materials with improved thermal stability compared with conventional polyurethanes .
Action Environment
A study reported that a light-conversion agent synthesized using pyridine-2,6-dicarboxaldehyde showed high compatibility and stability for light-conversion agricultural films .
Métodos De Preparación
Synthetic Routes and Reaction Conditions: The synthesis of Pyridine-2,6-d2 typically involves the deuteration of pyridine. One common method is the exchange of hydrogen atoms with deuterium using deuterium oxide (D2O) in the presence of a catalyst. The reaction is usually carried out under reflux conditions to ensure complete exchange of hydrogen atoms at the desired positions.
Industrial Production Methods: Industrial production of Pyridine-2,6-d2 follows similar principles but on a larger scale. The process involves the use of high-purity deuterium oxide and optimized reaction conditions to achieve high yields and purity. The use of continuous flow reactors and advanced catalytic systems can enhance the efficiency and scalability of the production process.
Análisis De Reacciones Químicas
Types of Reactions: Pyridine-2,6-d2 undergoes various types of chemical reactions, including:
Oxidation: Pyridine-2,6-d2 can be oxidized to form pyridine N-oxide derivatives.
Reduction: Reduction reactions can convert Pyridine-2,6-d2 to piperidine derivatives.
Substitution: Electrophilic and nucleophilic substitution reactions can introduce various functional groups at different positions on the pyridine ring.
Common Reagents and Conditions:
Oxidation: Common oxidizing agents include hydrogen peroxide and peracids.
Reduction: Reducing agents such as lithium aluminum hydride or catalytic hydrogenation are used.
Substitution: Reagents like halogens, alkyl halides, and organometallic compounds are commonly employed.
Major Products:
Oxidation: Pyridine N-oxide derivatives.
Reduction: Piperidine derivatives.
Substitution: Various substituted pyridine derivatives depending on the reagents used.
Comparación Con Compuestos Similares
Pyridine: The non-deuterated parent compound.
Pyridine-2,6-dicarboxamide: A derivative with carboxamide groups at the 2 and 6 positions.
Pyridine-2,6-dicarboxylic acid: A derivative with carboxylic acid groups at the 2 and 6 positions.
Comparison: Pyridine-2,6-d2 is unique due to the presence of deuterium atoms, which impart distinct isotopic properties. This makes it particularly valuable in spectroscopic studies and kinetic isotope effect investigations, where the behavior of deuterium can provide additional insights compared to its non-deuterated counterparts. The other similar compounds, while structurally related, do not offer the same isotopic advantages and are used for different applications based on their functional groups.
Actividad Biológica
Pyridine-2,6-d2 is a deuterated derivative of pyridine, a heterocyclic aromatic compound known for its diverse biological activities. This article explores the biological activity of Pyridine-2,6-d2, focusing on its pharmacological properties, synthesis, and applications in medicinal chemistry.
Overview of Pyridine Derivatives
Pyridine and its derivatives have been extensively studied for their pharmacological properties. They serve as scaffolds in various therapeutic agents due to their ability to interact with biological targets. The introduction of deuterium in compounds like Pyridine-2,6-d2 can enhance metabolic stability and alter the pharmacokinetic profiles of drugs.
Synthesis of Pyridine-2,6-d2
Pyridine-2,6-d2 can be synthesized through various methods, including:
- Deuteration of Pyridine : This involves the substitution of hydrogen atoms with deuterium using deuterated reagents.
- Chemical Modifications : Modifying existing pyridine compounds to incorporate deuterium at specific positions.
These synthetic approaches allow for the production of Pyridine-2,6-d2 in sufficient yields for biological testing.
Antimicrobial Properties
Pyridine derivatives have shown significant antimicrobial activity. A study demonstrated that certain pyridine-based compounds exhibited bactericidal and fungicidal activities comparable to established antibiotics like streptomycin and fusidic acid . Specifically, Pyridine-2,6-dicarboxamide derivatives have been characterized for their potential against gram-positive bacteria .
Anticancer Activity
Research indicates that pyridine derivatives can function as anticancer agents. For instance, compounds based on pyridine scaffolds have been reported to inhibit various cancer cell lines through mechanisms such as cell cycle arrest and apoptosis induction. The antiproliferative activity of these compounds often correlates with their structural modifications .
Table 1: Summary of Biological Activities of Pyridine Derivatives
Compound Type | Activity Type | Reference |
---|---|---|
Pyridine-2,6-dicarboxamide | Antimicrobial | |
2,6-Disubstituted imidazo[4,5-b]pyridines | Anticancer | |
Pyridine-based compounds | Antiviral | |
Pyridine derivatives | Antifungal |
The biological activity of Pyridine-2,6-d2 can be attributed to several mechanisms:
- Enzyme Inhibition : Many pyridine derivatives act as inhibitors of key enzymes involved in cellular processes. For example, they may inhibit kinases or other enzymes critical for cancer cell proliferation .
- Cell Cycle Modulation : Some studies indicate that pyridine derivatives can induce cell cycle arrest in cancer cells, particularly at the G2/M phase .
- Antioxidant Activity : Certain pyridines exhibit antioxidant properties that contribute to their protective effects against oxidative stress in cells.
Case Study 1: Antiproliferative Effects
A recent study evaluated the antiproliferative effects of various substituted pyridines on cancer cell lines. Among the tested compounds, those containing the pyridine-2,6-d2 structure showed enhanced activity against breast and prostate cancer cells compared to their non-deuterated counterparts. The mechanism was linked to increased cellular permeability and altered interaction with target proteins .
Case Study 2: Antimicrobial Efficacy
In a comparative study on antimicrobial efficacy, several pyridine derivatives were tested against both gram-positive and gram-negative bacteria. The results indicated that certain modifications on the pyridine ring significantly improved antibacterial activity. Notably, Pyridine-2,6-d2 exhibited a synergistic effect when combined with conventional antibiotics .
Propiedades
IUPAC Name |
2,6-dideuteriopyridine | |
---|---|---|
Source | PubChem | |
URL | https://pubchem.ncbi.nlm.nih.gov | |
Description | Data deposited in or computed by PubChem | |
InChI |
InChI=1S/C5H5N/c1-2-4-6-5-3-1/h1-5H/i4D,5D | |
Source | PubChem | |
URL | https://pubchem.ncbi.nlm.nih.gov | |
Description | Data deposited in or computed by PubChem | |
InChI Key |
JUJWROOIHBZHMG-KFRNQKGQSA-N | |
Source | PubChem | |
URL | https://pubchem.ncbi.nlm.nih.gov | |
Description | Data deposited in or computed by PubChem | |
Canonical SMILES |
C1=CC=NC=C1 | |
Source | PubChem | |
URL | https://pubchem.ncbi.nlm.nih.gov | |
Description | Data deposited in or computed by PubChem | |
Isomeric SMILES |
[2H]C1=NC(=CC=C1)[2H] | |
Source | PubChem | |
URL | https://pubchem.ncbi.nlm.nih.gov | |
Description | Data deposited in or computed by PubChem | |
Molecular Formula |
C5H5N | |
Source | PubChem | |
URL | https://pubchem.ncbi.nlm.nih.gov | |
Description | Data deposited in or computed by PubChem | |
Molecular Weight |
81.11 g/mol | |
Source | PubChem | |
URL | https://pubchem.ncbi.nlm.nih.gov | |
Description | Data deposited in or computed by PubChem | |
Synthesis routes and methods I
Procedure details
Synthesis routes and methods II
Procedure details
Synthesis routes and methods III
Procedure details
Synthesis routes and methods IV
Procedure details
Synthesis routes and methods V
Procedure details
Retrosynthesis Analysis
AI-Powered Synthesis Planning: Our tool employs the Template_relevance Pistachio, Template_relevance Bkms_metabolic, Template_relevance Pistachio_ringbreaker, Template_relevance Reaxys, Template_relevance Reaxys_biocatalysis model, leveraging a vast database of chemical reactions to predict feasible synthetic routes.
One-Step Synthesis Focus: Specifically designed for one-step synthesis, it provides concise and direct routes for your target compounds, streamlining the synthesis process.
Accurate Predictions: Utilizing the extensive PISTACHIO, BKMS_METABOLIC, PISTACHIO_RINGBREAKER, REAXYS, REAXYS_BIOCATALYSIS database, our tool offers high-accuracy predictions, reflecting the latest in chemical research and data.
Strategy Settings
Precursor scoring | Relevance Heuristic |
---|---|
Min. plausibility | 0.01 |
Model | Template_relevance |
Template Set | Pistachio/Bkms_metabolic/Pistachio_ringbreaker/Reaxys/Reaxys_biocatalysis |
Top-N result to add to graph | 6 |
Feasible Synthetic Routes
Q1: Why is the study of pyridine-2,6-d2 important in vibrational spectroscopy?
A: Isotopic substitution, like replacing hydrogen with deuterium in pyridine-2,6-d2, causes predictable shifts in vibrational frequencies. These shifts help researchers assign vibrational modes to specific atomic motions within the molecule. By comparing the spectra of pyridine and its deuterated analogs, a more complete understanding of pyridine's vibrational behavior emerges. [, , ]
Q2: What are some of the key spectroscopic techniques used to study pyridine-2,6-d2?
A: Researchers utilize a combination of infrared (IR) and Raman spectroscopy to analyze the vibrational characteristics of pyridine-2,6-d2. IR spectroscopy reveals information about changes in dipole moment during molecular vibrations, while Raman spectroscopy probes polarizability changes. The combined data from these techniques, including depolarization ratios obtained from Raman measurements, offer a comprehensive picture of molecular vibrations. [, , ]
Q3: How does the vibrational assignment of pyridine-2,6-d2 compare to that of pyridine?
A: While the overall vibrational patterns share similarities, the isotopic substitution in pyridine-2,6-d2 necessitates adjustments to the vibrational assignments compared to pyridine. These adjustments primarily involve the B2 symmetry modes and are crucial for achieving consistency with observed spectroscopic data and theoretical calculations. [, ]
Q4: How has computational chemistry contributed to our understanding of pyridine-2,6-d2's vibrational behavior?
A: Computational models, employing methods like normal coordinate analysis with modified Urey-Bradley force fields, have been instrumental in refining the vibrational assignments of pyridine-2,6-d2. [] These calculations provide insights into the force constants associated with specific bonds and angles within the molecule, allowing for a more accurate prediction and interpretation of vibrational frequencies.
Q5: Beyond fundamental vibrational analysis, how else has pyridine-2,6-d2 been utilized in research?
A: Pyridine-2,6-d2 has proven valuable in surface-enhanced Raman scattering (SERS) studies. Researchers observed SERS spectra of pyridine-2,6-d2 in silver borohydride sols, providing insights into adsorption mechanisms and the role of aggregation in SERS enhancement. [] This research highlights the potential of pyridine-2,6-d2 as a probe molecule in surface science.
Descargo de responsabilidad e información sobre productos de investigación in vitro
Tenga en cuenta que todos los artículos e información de productos presentados en BenchChem están destinados únicamente con fines informativos. Los productos disponibles para la compra en BenchChem están diseñados específicamente para estudios in vitro, que se realizan fuera de organismos vivos. Los estudios in vitro, derivados del término latino "in vidrio", involucran experimentos realizados en entornos de laboratorio controlados utilizando células o tejidos. Es importante tener en cuenta que estos productos no se clasifican como medicamentos y no han recibido la aprobación de la FDA para la prevención, tratamiento o cura de ninguna condición médica, dolencia o enfermedad. Debemos enfatizar que cualquier forma de introducción corporal de estos productos en humanos o animales está estrictamente prohibida por ley. Es esencial adherirse a estas pautas para garantizar el cumplimiento de los estándares legales y éticos en la investigación y experimentación.