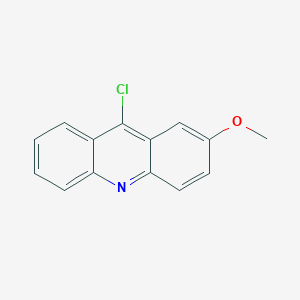
9-Chloro-2-methoxyacridine
Descripción general
Descripción
Métodos De Preparación
Synthetic Routes and Reaction Conditions: The synthesis of 9-Chloro-2-methoxyacridine typically involves the reaction of 6,9-dichloro-2-methoxyacridine with phenol and ammonium carbonate. This reaction yields 6-chloro-2-methoxy-acridin-9-ylamine, which can be further processed to obtain this compound .
Industrial Production Methods: In industrial settings, the production of this compound may involve large-scale synthesis using similar reaction conditions but optimized for higher yields and purity. The use of automated reactors and continuous flow systems can enhance the efficiency of the production process.
Análisis De Reacciones Químicas
Types of Reactions: 9-Chloro-2-methoxyacridine undergoes various chemical reactions, including:
Substitution Reactions: The chlorine atom at the ninth position can be substituted with other nucleophiles.
Oxidation and Reduction Reactions: The compound can participate in redox reactions, altering its oxidation state.
Intercalation with DNA: It forms complexes with DNA, which can be studied using various spectroscopic techniques.
Common Reagents and Conditions:
Substitution Reactions: Common reagents include nucleophiles such as amines and thiols.
Oxidation Reactions: Oxidizing agents like potassium permanganate or hydrogen peroxide can be used.
Reduction Reactions: Reducing agents such as sodium borohydride or lithium aluminum hydride are commonly employed.
Major Products Formed:
Substitution Products: Depending on the nucleophile used, various substituted acridines can be formed.
Oxidation Products: Oxidized derivatives of this compound.
Reduction Products: Reduced forms of the compound with altered functional groups.
Aplicaciones Científicas De Investigación
Fluorescent Probing and Membrane Studies
ACMA is widely utilized as a fluorescent probe to study energy transduction processes across biological membranes. Research involving the cyanobacterium Plectonema boryanum demonstrated that ACMA can effectively monitor both light-driven electron transfer and dark endogenous respiration within thylakoid membranes. The compound's fluorescence response is sensitive to various inhibitors, indicating its utility in exploring membrane dynamics and proton gradients .
Key Findings:
- Fluorescence Behavior: ACMA exhibits dualistic fluorescence behavior, with quenching and enhancement observed under different conditions.
- Proton Gradient Sensitivity: The compound's fluorescence is quenched when a pH gradient forms, making it a valuable tool for studying proton-pumping activities of membrane-bound ATPases .
DNA Intercalation
9-Chloro-2-methoxyacridine is known for its ability to intercalate into DNA, specifically binding to poly(dA-dT) sequences. This property makes it a significant compound in molecular biology research, particularly in studies involving DNA structure and function.
Applications:
- Cell Permeability: ACMA's ability to cross cell membranes allows it to be used in live-cell imaging studies to investigate cellular processes involving DNA.
- Fluorescent Detection: The excitation/emission maxima of ACMA (∼419/483 nm) enable its use with various UV-light sources, facilitating compatibility with other fluorescent dyes .
Inhibition of Enzymatic Activity
Recent studies have explored the synthesis of derivatives of 6-chloro-2-methoxyacridine linked to aryl triazole groups, evaluating their inhibitory effects on α-glucosidase. This enzyme plays a critical role in carbohydrate metabolism, making these derivatives potential candidates for managing type 2 diabetes mellitus.
Research Insights:
- Potency Evaluation: A series of synthesized compounds demonstrated varying degrees of α-glucosidase inhibition, with some derivatives exhibiting significantly better potency than the clinically used drug acarbose.
- Mechanistic Studies: Kinetic studies revealed competitive inhibition behavior for the most potent derivative, providing insights into the interaction mechanism at the molecular level .
Data Table: α-Glucosidase Inhibition Potency
Compound | IC50 (µM) | Comparison to Acarbose |
---|---|---|
7a | 169.1 ± 0.9 | Significantly more potent |
7b | >750 | Unfavorable substitution |
7c | >750 | Unfavorable substitution |
7h | 98 | Eight-fold better than acarbose |
Potential Therapeutic Applications
The diverse applications of this compound extend into therapeutic realms due to its biological activity against various targets. Its derivatives are being investigated for potential anticancer properties and their ability to inhibit specific enzymes involved in metabolic disorders.
Case Studies:
Mecanismo De Acción
The primary mechanism of action of 9-Chloro-2-methoxyacridine involves its ability to intercalate into DNA. This intercalation disrupts the normal structure of DNA, leading to inhibition of DNA replication and transcription. The compound selectively binds to poly(dA-dT) sequences, and its fluorescence properties change upon binding . Additionally, it can inhibit enzymes such as acetylcholinesterase, contributing to its biological effects .
Comparación Con Compuestos Similares
Chemical Identity :
- IUPAC Name : 9-Chloro-2-methoxyacridine
- Molecular Formula: C₁₄H₁₀ClNO
- Molecular Weight : 243.69 g/mol
- CAS Registry Number: Not explicitly listed in evidence, but structurally related compounds (e.g., 6,9-dichloro-2-methoxyacridine) are cited as CAS 86-38-4 .
Synthesis: this compound (C2) is synthesized via halogenation and methoxylation of acridine derivatives. reports a 77% yield using procedure C, producing a light green powder with a melting point (mp) of 153–155°C . It serves as a precursor for antileukemic agents and aminoacridine derivatives .
Table 1: Structural and Physical Properties
Key Differences :
Substitution Patterns :
- 9-Chloroacridine : Lacks methoxy/methyl groups, making it less reactive in coupling reactions compared to this compound .
- 6,9-Dichloro-2-methoxyacridine : Additional chlorine at position 6 increases molecular weight (278.13 vs. 243.69) and melting point (163–165°C vs. 153–155°C), enhancing its utility in synthesizing polyamine derivatives .
Reactivity :
- The methoxy group in this compound facilitates nucleophilic substitution at position 9, enabling Suzuki-Miyaura coupling for aryl-acridine hybrids .
- 9-Chloro-2-methylacridine’s methyl group improves solubility in organic solvents, favoring alkylation reactions .
Biological Activity: this compound derivatives exhibit antileukemic activity, while 6,9-dichloro-2-methoxyacridine is used in aminoquinoline-based antimalarials . Fluorinated analogues (e.g., 9-Chloro-8-fluoro-7-methyl-tetrahydroacridine) show enhanced pharmacokinetic profiles due to fluorine’s electronegativity .
Research Findings and Industrial Relevance
- Pharmaceutical Synthesis: this compound is critical in preparing 9-aminoacridines, which show promise in cancer therapy and enzyme inhibition .
- Analytical Chemistry : Used as a chromogenic agent for spectrophotometric determination of aspirin, with a detection limit of 6.38 µg/mL .
- Industrial Availability : 6,9-Dichloro-2-methoxyacridine is commercially available (Thermo Scientific, 98% purity) but listed as discontinued by CymitQuimica .
Actividad Biológica
9-Chloro-2-methoxyacridine is a compound of significant interest in medicinal chemistry and biochemistry due to its diverse biological activities. This article explores its mechanisms of action, biochemical properties, and potential applications in various fields, particularly in the treatment of parasitic infections and cancer.
Target Interactions
The primary target of this compound is DNA, specifically the poly(dA-dT) sequences. The compound intercalates into DNA, leading to structural alterations that can inhibit essential cellular processes such as DNA replication and transcription. This intercalation is facilitated by the compound's ability to form stable complexes with DNA, which can be detected through various spectroscopic techniques .
Biochemical Pathways
The interaction of this compound with cellular membranes and DNA affects several biochemical pathways. For instance, it has been shown to influence vacuolar pH and ion transport mechanisms within cells. The fluorescence properties of the compound are also pH-dependent, indicating that its efficacy may vary with changes in the cellular environment.
Cellular Effects
This compound exhibits notable cytotoxic effects against various cell lines, including those associated with parasitic infections. In vitro studies have demonstrated its antiproliferative properties against Leishmania species and other pathogens . The compound's effectiveness can vary based on the structural modifications at the 7-position of the acridine ring, highlighting the importance of chemical structure in determining biological activity.
Antiparasitic Activity
Research has shown that this compound and its derivatives possess significant antileishmanial and antimalarial activities. For example, studies reported IC50 values for certain derivatives ranging from 30.8 nM to 68.1 nM against different strains of Plasmodium falciparum, indicating potent activity compared to traditional treatments .
Anticancer Properties
In addition to its antiparasitic effects, this compound has been investigated for potential anticancer properties. Its ability to induce apoptosis in cancer cells through DNA intercalation and subsequent disruption of cellular processes has been documented. This mechanism positions it as a candidate for further development in cancer therapeutics .
Case Studies
-
Antileishmanial Activity
A study evaluated various acridine derivatives, including this compound, against Leishmania infantum. The results indicated that certain substitutions enhanced activity while maintaining low cytotoxicity to human cells. The study emphasized the need for structural optimization to improve therapeutic efficacy . -
Fluorescent Probes in Cellular Studies
The compound has also been utilized as a fluorescent probe in studies investigating energy transduction in cyanobacterial membranes. The findings revealed that this compound could effectively monitor changes in membrane potential and metabolic activity, showcasing its versatility beyond direct therapeutic applications .
Summary of Biological Activities
Activity Type | Target Organism/Cell Type | IC50 Value (nM) | Notes |
---|---|---|---|
Antileishmanial | Leishmania infantum | Varies by derivative | Structural modifications enhance activity |
Antimalarial | Plasmodium falciparum | 30.8 - 68.1 | Potent against resistant strains |
Anticancer | Various cancer cell lines | Varies | Induces apoptosis through DNA intercalation |
Fluorescent probe | Cyanobacteria | N/A | Monitors membrane potential changes |
Q & A
Basic Research Questions
Q. What are the standard protocols for synthesizing 9-chloro-2-methoxyacridine, and how can purity be verified?
- Methodological Answer : Synthesis typically involves chlorination of 2-methoxyacridine using reagents like phosphorus oxychloride (POCl₃) under reflux. Post-reaction, the product is purified via recrystallization or column chromatography. Purity verification requires analytical techniques such as high-performance liquid chromatography (HPLC) with UV detection and nuclear magnetic resonance (NMR) spectroscopy (¹H/¹³C) to confirm structural integrity .
Q. Which spectroscopic methods are most reliable for characterizing this compound?
- Methodological Answer : UV-Vis spectroscopy (200–400 nm) is effective for detecting conjugated π-systems, while mass spectrometry (MS) confirms molecular weight. Infrared (IR) spectroscopy identifies functional groups (e.g., C-Cl stretch at ~550–750 cm⁻¹). Cross-validation with NMR (e.g., methoxy group signals at δ 3.8–4.0 ppm) ensures structural accuracy .
Q. How can researchers ensure reproducibility in synthesizing derivatives of this compound?
- Methodological Answer : Document reaction parameters (temperature, solvent, stoichiometry) meticulously. Use controlled environments (e.g., inert gas for moisture-sensitive steps) and validate each intermediate via thin-layer chromatography (TLC). Reference protocols from peer-reviewed journals and replicate published procedures as a baseline .
Advanced Research Questions
Q. How can contradictory spectral data in this compound derivatives be resolved?
- Methodological Answer : Contradictions may arise from impurities or tautomeric forms. Employ high-resolution mass spectrometry (HRMS) to confirm molecular formulas. Use 2D NMR (e.g., COSY, HSQC) to resolve overlapping signals. Computational tools like density functional theory (DFT) can predict spectral patterns for comparison .
Q. What strategies optimize the photostability of this compound in experimental applications?
- Methodological Answer : Test under varying light conditions (UV/visible) and monitor degradation via HPLC. Incorporate stabilizers like antioxidants (e.g., BHT) or UV absorbers. Evaluate solvent effects—polar aprotic solvents (e.g., DMSO) may reduce photodegradation compared to protic ones .
Q. How can computational modeling predict the reactivity of this compound in nucleophilic substitution reactions?
- Methodological Answer : Use software like Gaussian or ORCA to calculate frontier molecular orbitals (HOMO/LUMO) and electrostatic potential maps. Molecular dynamics simulations can model reaction pathways, while QSAR models correlate substituent effects with reaction rates .
Q. What are the challenges in crystallizing this compound, and how can they be addressed?
- Methodological Answer : Poor crystallization may stem from molecular flexibility. Use slow evaporation with mixed solvents (e.g., dichloromethane/hexane) or employ diffusion methods. Single-crystal X-ray diffraction (SCXRD) requires high-quality crystals; consider co-crystallization agents or temperature-controlled setups .
Q. Data Analysis & Interpretation
Q. How should researchers handle outliers in biological activity assays involving this compound analogs?
- Methodological Answer : Apply statistical tests (e.g., Grubbs’ test) to identify outliers. Replicate experiments to distinguish between experimental error and genuine biological variability. Use dose-response curves (IC₅₀/EC₅₀) with confidence intervals to assess significance .
Q. What metrics validate the accuracy of quantitative analyses (e.g., spectrophotometric determination) for this compound?
- Methodological Answer : Calibrate instruments with certified reference materials. Calculate limits of detection (LOD) and quantification (LOQ) via signal-to-noise ratios. Validate methods using spike-and-recovery experiments and inter-laboratory comparisons .
Q. Experimental Design & Troubleshooting
Q. How can researchers design a robust structure-activity relationship (SAR) study for this compound derivatives?
- Methodological Answer : Systematically vary substituents (e.g., halogens, methoxy groups) and test against a consistent biological target. Use multivariate analysis (e.g., PCA) to identify key structural contributors. Include positive/negative controls and blind assays to minimize bias .
Q. What are common pitfalls in scaling up this compound synthesis, and how can they be mitigated?
- Methodological Answer : Heat dissipation and mixing efficiency often limit scalability. Use jacketed reactors for temperature control and optimize stirring rates. Pilot studies in batch reactors can identify critical parameters (e.g., exothermic peaks) before transitioning to flow chemistry .
Q. Literature & Reproducibility
Q. How can researchers critically evaluate conflicting literature reports on the biological efficacy of this compound?
- Methodological Answer : Compare experimental conditions (e.g., cell lines, assay protocols) across studies. Replicate key experiments with standardized controls. Use meta-analysis tools to aggregate data and identify trends obscured by variability .
Q. What steps ensure compliance with ethical and reproducibility standards when publishing this compound research?
- Methodological Answer : Adhere to FAIR principles (Findable, Accessible, Interoperable, Reusable). Deposit raw data in repositories like Zenodo. Include detailed supplementary materials (e.g., NMR spectra, crystallographic data) and cite primary literature to avoid plagiarism .
Propiedades
IUPAC Name |
9-chloro-2-methoxyacridine | |
---|---|---|
Source | PubChem | |
URL | https://pubchem.ncbi.nlm.nih.gov | |
Description | Data deposited in or computed by PubChem | |
InChI |
InChI=1S/C14H10ClNO/c1-17-9-6-7-13-11(8-9)14(15)10-4-2-3-5-12(10)16-13/h2-8H,1H3 | |
Source | PubChem | |
URL | https://pubchem.ncbi.nlm.nih.gov | |
Description | Data deposited in or computed by PubChem | |
InChI Key |
BGAMCDWSLKLEJQ-UHFFFAOYSA-N | |
Source | PubChem | |
URL | https://pubchem.ncbi.nlm.nih.gov | |
Description | Data deposited in or computed by PubChem | |
Canonical SMILES |
COC1=CC2=C(C3=CC=CC=C3N=C2C=C1)Cl | |
Source | PubChem | |
URL | https://pubchem.ncbi.nlm.nih.gov | |
Description | Data deposited in or computed by PubChem | |
Molecular Formula |
C14H10ClNO | |
Source | PubChem | |
URL | https://pubchem.ncbi.nlm.nih.gov | |
Description | Data deposited in or computed by PubChem | |
DSSTOX Substance ID |
DTXSID20497615 | |
Record name | 9-Chloro-2-methoxyacridine | |
Source | EPA DSSTox | |
URL | https://comptox.epa.gov/dashboard/DTXSID20497615 | |
Description | DSSTox provides a high quality public chemistry resource for supporting improved predictive toxicology. | |
Molecular Weight |
243.69 g/mol | |
Source | PubChem | |
URL | https://pubchem.ncbi.nlm.nih.gov | |
Description | Data deposited in or computed by PubChem | |
CAS No. |
16492-13-0 | |
Record name | 9-Chloro-2-methoxyacridine | |
Source | EPA DSSTox | |
URL | https://comptox.epa.gov/dashboard/DTXSID20497615 | |
Description | DSSTox provides a high quality public chemistry resource for supporting improved predictive toxicology. | |
Retrosynthesis Analysis
AI-Powered Synthesis Planning: Our tool employs the Template_relevance Pistachio, Template_relevance Bkms_metabolic, Template_relevance Pistachio_ringbreaker, Template_relevance Reaxys, Template_relevance Reaxys_biocatalysis model, leveraging a vast database of chemical reactions to predict feasible synthetic routes.
One-Step Synthesis Focus: Specifically designed for one-step synthesis, it provides concise and direct routes for your target compounds, streamlining the synthesis process.
Accurate Predictions: Utilizing the extensive PISTACHIO, BKMS_METABOLIC, PISTACHIO_RINGBREAKER, REAXYS, REAXYS_BIOCATALYSIS database, our tool offers high-accuracy predictions, reflecting the latest in chemical research and data.
Strategy Settings
Precursor scoring | Relevance Heuristic |
---|---|
Min. plausibility | 0.01 |
Model | Template_relevance |
Template Set | Pistachio/Bkms_metabolic/Pistachio_ringbreaker/Reaxys/Reaxys_biocatalysis |
Top-N result to add to graph | 6 |
Feasible Synthetic Routes
Descargo de responsabilidad e información sobre productos de investigación in vitro
Tenga en cuenta que todos los artículos e información de productos presentados en BenchChem están destinados únicamente con fines informativos. Los productos disponibles para la compra en BenchChem están diseñados específicamente para estudios in vitro, que se realizan fuera de organismos vivos. Los estudios in vitro, derivados del término latino "in vidrio", involucran experimentos realizados en entornos de laboratorio controlados utilizando células o tejidos. Es importante tener en cuenta que estos productos no se clasifican como medicamentos y no han recibido la aprobación de la FDA para la prevención, tratamiento o cura de ninguna condición médica, dolencia o enfermedad. Debemos enfatizar que cualquier forma de introducción corporal de estos productos en humanos o animales está estrictamente prohibida por ley. Es esencial adherirse a estas pautas para garantizar el cumplimiento de los estándares legales y éticos en la investigación y experimentación.