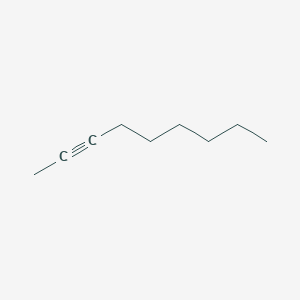
2-Nonino
Descripción general
Descripción
2-Nonyne is an organic compound that falls under the category of alkynes, which are hydrocarbons containing at least one carbon-carbon triple bond. The molecular formula for 2-Nonyne is C₉H₁₆, indicating that it consists of nine carbon atoms and sixteen hydrogen atoms. This compound is a colorless liquid at room temperature and has a characteristic sharp or pungent smell, typical of many alkynes .
Aplicaciones Científicas De Investigación
2-Nonyne is primarily utilized in the research and development sectors of chemistry. It serves as a building block in the synthesis of more complex molecules for various purposes, including:
Chemistry: Used in the synthesis of polymers and other organic compounds.
Biology: Employed in the study of enzyme mechanisms and metabolic pathways.
Medicine: Investigated for its potential use in the development of pharmaceuticals.
Industry: Utilized in the production of specialty chemicals and materials.
Mecanismo De Acción
Target of Action
2-Nonyne is an organic compound that belongs to the class of alkynes . Alkynes are characterized by the presence of a carbon-carbon triple bond, which is the functional group of these molecules
Mode of Action
As an alkyne, it contains a carbon-carbon triple bond, which is a region of high electron density and can participate in various chemical reactions . This property could potentially allow 2-Nonyne to interact with various biological targets, leading to different biochemical effects.
Pharmacokinetics
The molecular weight of 2-Nonyne is 124.2233 , which could potentially influence its bioavailability and distribution within the body
Result of Action
As an alkyne, 2-Nonyne has a carbon-carbon triple bond, which could potentially interact with various biological targets and lead to different cellular effects . .
Métodos De Preparación
Synthetic Routes and Reaction Conditions: The synthesis of 2-Nonyne is typically carried out in a laboratory setting using organic chemistry methods. The most common approach involves a procedure known as dehydrohalogenation. Initially, a 1,2-dihaloalkane is reacted with a strong base such as sodium amide (NaNH₂) or potassium hydroxide (KOH). This reaction leads to the formation of an alkyne through the elimination of a hydrogen halide (HX) molecule from the dihaloalkane, resulting in the formation of a triple bond between two carbon atoms .
Industrial Production Methods: While specific industrial production methods for 2-Nonyne are not extensively documented, the general principles of alkyne synthesis apply. Industrial production would likely involve large-scale dehydrohalogenation reactions using efficient catalysts and optimized reaction conditions to maximize yield and purity.
Análisis De Reacciones Químicas
Types of Reactions: 2-Nonyne, like other alkynes, undergoes a variety of chemical reactions due to the presence of its carbon-carbon triple bond. These reactions include:
Hydrogenation: Addition of hydrogen to form alkanes.
Halogenation: Addition of halogens such as chlorine or bromine.
Hydration: Addition of water to form alcohols.
Oxidation: Reaction with oxidizing agents to form carboxylic acids or ketones.
Reduction: Reaction with reducing agents to form alkenes or alkanes.
Common Reagents and Conditions:
Hydrogenation: Typically carried out using hydrogen gas (H₂) and a metal catalyst such as palladium on carbon (Pd/C).
Halogenation: Involves halogens like chlorine (Cl₂) or bromine (Br₂) in the presence of a solvent such as carbon tetrachloride (CCl₄).
Hydration: Often performed using water (H₂O) and an acid catalyst like sulfuric acid (H₂SO₄).
Oxidation: Common oxidizing agents include potassium permanganate (KMnO₄) or ozone (O₃).
Reduction: Reducing agents such as lithium aluminum hydride (LiAlH₄) or sodium borohydride (NaBH₄) are used.
Major Products Formed:
Hydrogenation: Nonane (C₉H₂₀)
Halogenation: 2,2-Dihalononane
Hydration: 2-Nonanol
Oxidation: Nonanoic acid or 2-Nonanone
Reduction: 2-Nonene or Nonane.
Comparación Con Compuestos Similares
1-Nonyne (C₉H₁₆): Another alkyne with a triple bond at the first carbon atom.
2-Octyne (C₈H₁₄): An alkyne with a similar structure but one less carbon atom.
2-Decyne (C₁₀H₁₈): An alkyne with a similar structure but one more carbon atom.
Uniqueness of 2-Nonyne: 2-Nonyne is unique due to its specific position of the triple bond at the second carbon atom, which influences its reactivity and the types of reactions it can undergo. This positional isomerism differentiates it from other nonynes and alkynes, providing distinct chemical properties and applications .
Actividad Biológica
2-Nonyne, a linear alkyne with the chemical formula C₉H₁₈, has garnered attention in various fields of research due to its unique structural properties and potential biological activities. This article explores the biological activity of 2-nonyne, including its antimicrobial properties, applications in bioorthogonal chemistry, and relevant case studies.
Chemical Structure and Properties
2-Nonyne is characterized by a triple bond between the second and third carbon atoms in a nine-carbon chain. This unsaturation contributes to its reactivity and potential biological applications. The compound is often utilized as a building block in organic synthesis and has implications in medicinal chemistry.
Biological Activity
1. Antimicrobial Properties
Recent studies have investigated the antimicrobial activity of various alkynes, including 2-nonyne. A study focused on the phytochemical analysis of plants revealed that certain alkyne derivatives exhibited significant antimicrobial effects against various bacterial strains. Although specific data on 2-nonyne's activity is limited, related compounds have shown promising results.
Compound | Bacterial Strain | Minimum Inhibitory Concentration (MIC) |
---|---|---|
2-Nonyne | E. coli | Not specifically reported |
Related Alkyne | Staphylococcus aureus | 0.39 mg/mL |
This table illustrates the potential for similar compounds to exhibit significant biological activity, suggesting that 2-nonyne may also possess antimicrobial properties worth further exploration.
2. Bioorthogonal Chemistry Applications
2-Nonyne has been utilized in bioorthogonal reactions, particularly in the context of strain-promoted alkyne-azide cycloaddition (SPAAC). This reaction is pivotal for labeling biomolecules in living organisms without interfering with native biological processes. For instance, derivatives of 2-nonyne have been employed to conjugate therapeutic agents with targeting moieties, enhancing drug delivery systems.
Case Studies
Case Study 1: Antimicrobial Activity Analysis
In a comparative study examining the antimicrobial effects of various alkyne compounds, researchers found that certain derivatives displayed significant inhibition against pathogenic bacteria. Although direct studies on 2-nonyne are sparse, the findings suggest that its structural analogs could be effective against similar strains.
Case Study 2: Bioorthogonal Applications
A study published in ACS Publications highlighted the use of alkyne derivatives in bioorthogonal chemistry for conjugating antibodies to fluorophores. The efficiency of these reactions was demonstrated using various alkynes, including those structurally related to 2-nonyne. This application underscores the compound's potential utility in therapeutic contexts.
Propiedades
IUPAC Name |
non-2-yne | |
---|---|---|
Source | PubChem | |
URL | https://pubchem.ncbi.nlm.nih.gov | |
Description | Data deposited in or computed by PubChem | |
InChI |
InChI=1S/C9H16/c1-3-5-7-9-8-6-4-2/h3,5,7-9H2,1-2H3 | |
Source | PubChem | |
URL | https://pubchem.ncbi.nlm.nih.gov | |
Description | Data deposited in or computed by PubChem | |
InChI Key |
LXKRETAGISZJAD-UHFFFAOYSA-N | |
Source | PubChem | |
URL | https://pubchem.ncbi.nlm.nih.gov | |
Description | Data deposited in or computed by PubChem | |
Canonical SMILES |
CCCCCCC#CC | |
Source | PubChem | |
URL | https://pubchem.ncbi.nlm.nih.gov | |
Description | Data deposited in or computed by PubChem | |
Molecular Formula |
C9H16 | |
Source | PubChem | |
URL | https://pubchem.ncbi.nlm.nih.gov | |
Description | Data deposited in or computed by PubChem | |
DSSTOX Substance ID |
DTXSID10173077 | |
Record name | 2-Nonyne | |
Source | EPA DSSTox | |
URL | https://comptox.epa.gov/dashboard/DTXSID10173077 | |
Description | DSSTox provides a high quality public chemistry resource for supporting improved predictive toxicology. | |
Molecular Weight |
124.22 g/mol | |
Source | PubChem | |
URL | https://pubchem.ncbi.nlm.nih.gov | |
Description | Data deposited in or computed by PubChem | |
CAS No. |
19447-29-1 | |
Record name | 2-Nonyne | |
Source | ChemIDplus | |
URL | https://pubchem.ncbi.nlm.nih.gov/substance/?source=chemidplus&sourceid=0019447291 | |
Description | ChemIDplus is a free, web search system that provides access to the structure and nomenclature authority files used for the identification of chemical substances cited in National Library of Medicine (NLM) databases, including the TOXNET system. | |
Record name | 2-Nonyne | |
Source | EPA DSSTox | |
URL | https://comptox.epa.gov/dashboard/DTXSID10173077 | |
Description | DSSTox provides a high quality public chemistry resource for supporting improved predictive toxicology. | |
Record name | 2-Nonyne | |
Source | European Chemicals Agency (ECHA) | |
URL | https://echa.europa.eu/information-on-chemicals | |
Description | The European Chemicals Agency (ECHA) is an agency of the European Union which is the driving force among regulatory authorities in implementing the EU's groundbreaking chemicals legislation for the benefit of human health and the environment as well as for innovation and competitiveness. | |
Explanation | Use of the information, documents and data from the ECHA website is subject to the terms and conditions of this Legal Notice, and subject to other binding limitations provided for under applicable law, the information, documents and data made available on the ECHA website may be reproduced, distributed and/or used, totally or in part, for non-commercial purposes provided that ECHA is acknowledged as the source: "Source: European Chemicals Agency, http://echa.europa.eu/". Such acknowledgement must be included in each copy of the material. ECHA permits and encourages organisations and individuals to create links to the ECHA website under the following cumulative conditions: Links can only be made to webpages that provide a link to the Legal Notice page. | |
Retrosynthesis Analysis
AI-Powered Synthesis Planning: Our tool employs the Template_relevance Pistachio, Template_relevance Bkms_metabolic, Template_relevance Pistachio_ringbreaker, Template_relevance Reaxys, Template_relevance Reaxys_biocatalysis model, leveraging a vast database of chemical reactions to predict feasible synthetic routes.
One-Step Synthesis Focus: Specifically designed for one-step synthesis, it provides concise and direct routes for your target compounds, streamlining the synthesis process.
Accurate Predictions: Utilizing the extensive PISTACHIO, BKMS_METABOLIC, PISTACHIO_RINGBREAKER, REAXYS, REAXYS_BIOCATALYSIS database, our tool offers high-accuracy predictions, reflecting the latest in chemical research and data.
Strategy Settings
Precursor scoring | Relevance Heuristic |
---|---|
Min. plausibility | 0.01 |
Model | Template_relevance |
Template Set | Pistachio/Bkms_metabolic/Pistachio_ringbreaker/Reaxys/Reaxys_biocatalysis |
Top-N result to add to graph | 6 |
Feasible Synthetic Routes
Q1: What is the structural characterization of 2-Nonyne?
A1: 2-Nonyne is a linear aliphatic disubstituted acetylene with the molecular formula C9H16 and a molecular weight of 124.24 g/mol. While specific spectroscopic data wasn't provided in the provided research excerpts, its structure can be confirmed through techniques such as 1H NMR, 13C NMR, and IR spectroscopy.
Q2: How does the chain length of aliphatic disubstituted acetylenes like 2-Nonyne affect their polymerization?
A2: Research suggests that increasing the side-chain length in poly(2-alkylacetylenes) influences their gas permeation properties . Additionally, while 5-Dodecyne, with a triple bond further within the chain, polymerizes slower than 2-Nonyne, their living polymerization characteristics are similar . This suggests that while chain length and triple bond position can impact polymerization rate, the living character of the polymerization using a MoOCl4-n-Bu4Sn-EtOH catalyst system may be retained.
Q3: Can 2-Nonyne be used in the synthesis of block copolymers?
A3: Yes, 2-Nonyne can be used in the synthesis of block copolymers. Research has demonstrated the successful synthesis of diblock copolymers through the sequential living polymerization of internal linear alkynes, including 2-Nonyne . This suggests its potential use in creating more complex polymer structures with tailored properties. Additionally, studies highlight the synthesis of block copolymers containing 2-Nonyne alongside other monomers like 1-chloro-2-phenylacetylene and (p-n-butyl-o,o,m,m-tetrafluorophenyl)acetylene using MoOCl4-based catalysts . This further exemplifies the versatility of 2-Nonyne in block copolymer synthesis.
Q4: Has 2-Nonyne been identified in natural sources?
A4: Yes, 2-Nonyne has been identified as a constituent of the essential oil extracted from Mentha pulegium, also known as Pennyroyal, at different stages of its development . While it wasn't the major constituent, its presence contributes to the overall chemical profile of the essential oil.
Q5: What are the potential applications of polymers derived from 2-Nonyne?
A6: While specific applications of 2-Nonyne derived polymers aren't detailed in the provided research, its use in synthesizing block copolymers opens possibilities for various applications. Block copolymers are known for their ability to self-assemble into well-defined nanostructures, making them suitable for applications in drug delivery, nanomaterials, and other areas requiring controlled morphology and properties.
Descargo de responsabilidad e información sobre productos de investigación in vitro
Tenga en cuenta que todos los artículos e información de productos presentados en BenchChem están destinados únicamente con fines informativos. Los productos disponibles para la compra en BenchChem están diseñados específicamente para estudios in vitro, que se realizan fuera de organismos vivos. Los estudios in vitro, derivados del término latino "in vidrio", involucran experimentos realizados en entornos de laboratorio controlados utilizando células o tejidos. Es importante tener en cuenta que estos productos no se clasifican como medicamentos y no han recibido la aprobación de la FDA para la prevención, tratamiento o cura de ninguna condición médica, dolencia o enfermedad. Debemos enfatizar que cualquier forma de introducción corporal de estos productos en humanos o animales está estrictamente prohibida por ley. Es esencial adherirse a estas pautas para garantizar el cumplimiento de los estándares legales y éticos en la investigación y experimentación.