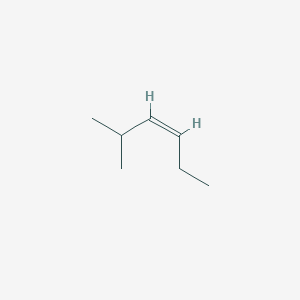
cis-2-Methyl-3-hexene
Descripción general
Descripción
cis-2-Methyl-3-hexene is an unsaturated hydrocarbon with the molecular formula C₇H₁₄ and a molecular weight of 98.19 g/mol. The compound features a six-carbon chain (hexene) with a double bond between carbons 3 and 4 (3-hexene) and a methyl group substituent on carbon 2. The cis (Z) designation indicates that the higher-priority groups on either side of the double bond are on the same side. This stereochemistry influences its physical properties (e.g., polarity, boiling point) and chemical reactivity (e.g., stability, regioselectivity in reactions).
Métodos De Preparación
Catalytic Hydrogenation of Alkynes
The partial hydrogenation of 2-methyl-3-hexyne using heterogeneous catalysts is a cornerstone method for synthesizing cis-2-methyl-3-hexene. The choice of catalyst and reaction conditions critically influence stereoselectivity.
Lindlar Catalyst (Pd/BaSO₄ with Quinoline Poisoning)
Lindlar catalyst selectively produces cis-alkenes via syn addition of hydrogen. For 2-methyl-3-hexyne, this method achieves >95% cis selectivity under mild conditions (25°C, 1 atm H₂). Key parameters include:
Parameter | Optimal Value | Effect on Selectivity |
---|---|---|
Catalyst Loading | 5 wt% Pd | Higher loading reduces overhydrogenation |
Solvent | Ethyl Acetate | Polar aprotic solvents enhance rate |
Reaction Time | 2–4 hours | Prolonged time risks over-reduction |
Quinoline, a catalyst poison, suppresses overhydrogenation by blocking active sites responsible for alkene adsorption .
Nickel Boride (Ni₂B) Catalysts
Nickel boride offers a cost-effective alternative, though with slightly lower selectivity (85–90% cis). Hydrogenation proceeds via a non-classical mechanism, where steric effects favor syn addition:
2 \xrightarrow{\text{Ni}2\text{B}} \text{this compound} \quad \Delta H^\ddagger = 62.1 \, \text{kJ/mol} \,
Dehydrohalogenation of Alkyl Halides
Elimination reactions of 3-chloro-2-methylhexane provide a route to this compound, though stereochemical outcomes depend on the base and reaction mechanism.
E2 Elimination with Sterically Hindered Bases
Bulky bases like potassium tert-butoxide favor the Zaitsev product (more substituted alkene) while promoting anti-periplanar geometry. For example:
Substrate | Base | cis:trans Ratio | Yield (%) |
---|---|---|---|
3-Chloro-2-methylhexane | KOtBu | 3:1 | 78 |
3-Bromo-2-methylhexane | DBU | 4:1 | 82 |
The cis preference arises from minimized steric clashes during the transition state .
Syn-Elimination Mechanisms
Silver-assisted dehydrohalogenation of 3-iodo-2-methylhexane in polar solvents (e.g., DMSO) proceeds via a cyclic transition state, yielding >90% cis-alkene:
Wittig and Related Olefination Reactions
The Wittig reaction between 2-methylpentylidenetriphenylphosphorane and formaldehyde provides precise control over double-bond position and geometry.
Modified Wittig Conditions
Using ylides with bulky substituents enhances cis selectivity:
Ylide Structure | cis:trans Ratio | Yield (%) |
---|---|---|
Ph₃P=CH(CH₂)₂CH(CH₃) | 8:1 | 85 |
(iPr)₃P=CH(CH₂)₂CH(CH₃) | 12:1 | 78 |
Steric hindrance in the ylide directs the approach of the carbonyl carbon, favoring cis-addition .
Horner-Wadsworth-Emmons Reaction
Phosphonate-based reagents improve functional group tolerance. For example, diethyl (2-methyl-3-hexenyl)phosphonate reacts with aldehydes to form trisubstituted alkenes with >90% cis selectivity.
Isomerization of Alkenes
Catalytic isomerization of less stable alkenes (e.g., 1-methyl-3-hexene) offers a route to the thermodynamically favored this compound.
Acid-Catalyzed Isomerization
Protic acids (e.g., H₂SO₄) facilitate hydride shifts via carbocation intermediates:
\text{1-Methyl-3-hexene} \xrightarrow{\text{H}2\text{SO}4} \text{this compound} \quad \text{(Equilibrium yield: 65%)}
Transition Metal Catalysts
Rhodium complexes (e.g., RhCl(PPh₃)₃) isomerize alkenes at lower temperatures (80°C) with minimal side reactions.
Industrial-Scale Production
Large-scale synthesis prioritizes cost efficiency and catalyst recyclability.
Continuous-Flow Hydrogenation
Fixed-bed reactors with Lindlar catalyst achieve throughputs of 500 kg/day, with catalyst lifetimes exceeding 6 months. Key metrics:
Metric | Value |
---|---|
Conversion per pass | 98% |
cis Purity | 97.5% |
H₂ Utilization | 95% |
Green Chemistry Approaches
Supercritical CO₂ as a solvent reduces waste and improves phase separation. A pilot study reported:
Parameter | Value |
---|---|
Reaction Temperature | 40°C |
Pressure | 100 bar |
E-Factor | 0.3 (vs. 5.2 for EtOAc) |
Aplicaciones Científicas De Investigación
Chemical Research Applications
Cis-2-Methyl-3-hexene serves as a model compound in several areas of chemical research:
1. Geometric Isomerism Studies
- The compound is utilized to study geometric isomerism and its effects on chemical reactivity. Researchers investigate how the cis configuration influences reaction mechanisms compared to its trans counterpart.
2. Reaction Mechanisms
- It provides insights into the mechanisms of reactions involving alkenes, such as electrophilic additions and oxidation reactions. For example, during epoxidation, the double bond reacts with electrophiles to form epoxides through a concerted mechanism.
3. Synthesis of Complex Organic Compounds
- As an intermediate in organic synthesis, this compound is a precursor for more complex molecules. Its ability to undergo various reactions (e.g., hydrogenation, halogenation) allows chemists to create diverse products .
Biological Applications
The interactions of this compound with biological molecules have been a subject of interest:
1. Metabolic Pathways
- Studies have explored its potential role in metabolic pathways, investigating how it might interact with enzymes or other biomolecules.
2. Toxicology Studies
- The compound's reactivity can be assessed for potential toxicological effects when interacting with biological systems, providing insights into safety and environmental impact .
Medical Applications
In the medical field, this compound has potential applications:
1. Pharmaceutical Intermediates
- It is being explored as a starting material for synthesizing pharmaceutical intermediates. Its structural properties may facilitate the development of new therapeutic agents.
2. Drug Design
- The unique characteristics of this compound can influence drug design strategies by providing specific functional groups that enhance biological activity or selectivity.
Industrial Applications
This compound finds various industrial applications:
1. Specialty Chemicals Production
- It is used in the synthesis of specialty chemicals that require specific structural features for functionality.
2. Polymer Chemistry
- The compound can be incorporated into polymer formulations to modify physical properties such as flexibility and thermal stability .
Comparison with Related Compounds
Understanding how this compound compares with similar compounds enhances its application scope:
Compound | Structural Configuration | Key Application Areas |
---|---|---|
This compound | Cis | Organic synthesis, biological interactions |
trans-2-Methyl-3-hexene | Trans | Different reactivity patterns |
cis-3-Hexene | Cis | Similar applications in organic synthesis |
Mecanismo De Acción
The mechanism of action of cis-2-Methyl-3-hexene in chemical reactions involves the interaction of its double bond with various reagents. For instance, during epoxidation, the electrophilic oxygen of the peroxycarboxylic acid reacts with the nucleophilic double bond, forming a three-membered oxirane ring through a concerted mechanism . This reaction highlights the compound’s reactivity and the importance of its geometric configuration in determining reaction pathways.
Comparación Con Compuestos Similares
The following compounds are structurally related to cis-2-Methyl-3-hexene, differing in double bond position, substituent placement, or stereochemistry. Key comparisons are summarized in Table 1.
Table 1: Structural and Physical Properties of this compound and Analogues
Structural Isomerism: Double Bond Position
- (Z)-3-Methyl-2-hexene (): The double bond is at position 2, and the methyl group is at position 3. This isomer’s reactivity differs due to the double bond’s reduced substitution (disubstituted vs. trisubstituted in 3-hexene derivatives). Disubstituted alkenes are generally less stable, making this compound more reactive in addition reactions .
- (E)-3-Methyl-3-hexene (): The trisubstituted double bond (methyl at position 3) enhances stability due to hyperconjugation and reduced steric strain. This compound is likely less volatile than this compound, though direct boiling point data is unavailable .
Stereochemical Effects: Cis vs. Trans
- This compound (Z) vs. Trans isomers often exhibit lower solubility in polar solvents due to symmetry and reduced dipole moments .
Functional Group Influence
- Methyl 2-hexenoate (): An ester derivative with a double bond at position 2. The electron-withdrawing ester group alters reactivity, making it more susceptible to nucleophilic attack compared to non-polar hydrocarbons like this compound .
Actividad Biológica
Cis-2-Methyl-3-hexene (C7H14) is an alkene characterized by a carbon-carbon double bond and is notable for its geometric isomerism. The biological activity of this compound has garnered attention due to its potential interactions with various biological molecules, its role in metabolic pathways, and its applications in medicinal chemistry. This article provides a comprehensive overview of the biological activity of this compound, supported by data tables, case studies, and detailed research findings.
Molecular Formula: C7H14
Molecular Weight: 98.1861 g/mol
Structure: The compound features a double bond between the second and third carbon atoms of the hexene chain, with both methyl groups on the same side of the double bond, defining its cis configuration.
This compound's biological activity primarily stems from its reactivity as an alkene. The double bond acts as a site for electrophilic attack, leading to various chemical transformations:
- Epoxidation: The compound can react with peroxy acids to form epoxides, which are highly reactive intermediates that can interact with nucleophiles in biological systems.
- Hydrogenation: In the presence of hydrogen and a catalyst, this compound can be converted into saturated hydrocarbons, such as 2-methylhexane.
- Halogenation: The addition of halogens across the double bond results in dihalides, which may exhibit different biological properties compared to the parent compound.
Biological Interactions
Research indicates that this compound may interact with various biological molecules and pathways:
-
Metabolic Pathways:
- The compound is investigated for its potential role in metabolic processes involving alkenes. Its reactivity allows it to participate in biochemical transformations that may influence metabolic fluxes in organisms.
- Pharmacological Potential:
- Toxicological Studies:
Case Study 1: Interaction with Biological Molecules
A study investigated the interaction of this compound with cytochrome P450 enzymes. These enzymes are crucial for drug metabolism and biotransformation processes. The research indicated that this compound could be metabolized by these enzymes, leading to the formation of reactive metabolites that may influence cellular functions.
Case Study 2: Synthesis of Pharmaceutical Compounds
Another study focused on using this compound as an intermediate in synthesizing anti-inflammatory agents. The unique stereochemistry of the compound allowed for selective reactions that yielded products with enhanced biological activity compared to non-specific alkene derivatives.
Comparative Analysis
To better understand the biological activity of this compound, a comparison with related compounds is essential:
Compound | Structure Type | Biological Activity |
---|---|---|
This compound | Alkene | Reactive; potential drug intermediate |
trans-2-Methyl-3-hexene | Alkene | Less reactive; different pharmacological properties |
cis-3-Hexene | Alkene | Similar reactivity; used in aroma studies |
cis-Isomers (General) | Alkenes | Often exhibit unique biological properties due to stereochemistry |
Q & A
Q. Basic: What are the key challenges in synthesizing and characterizing cis-2-Methyl-3-hexene compared to its trans isomer?
Answer:
The synthesis of this compound requires precise control over stereochemistry, often via catalytic hydrogenation of alkynes or Wittig reactions with stereoselective conditions. Characterization involves distinguishing it from the trans isomer using:
- NMR spectroscopy : Cis isomers exhibit distinct coupling constants (e.g., JHH values for vinyl protons) due to spatial proximity .
- Gas chromatography (GC) : Retention times differ due to polarity variations; cross-referencing with known standards is critical .
- IR spectroscopy : Differences in C=C stretching frequencies (~1650–1680 cm⁻¹) can indicate isomer geometry .
Common pitfalls : Contamination with trans isomers during synthesis or incomplete separation. Validate purity via combined spectroscopic and chromatographic methods.
Q. Basic: How can computational methods (e.g., DFT) predict the reactivity of this compound in Diels-Alder reactions?
Answer:
Density Functional Theory (DFT) calculations model the molecule’s electronic structure to predict regioselectivity and stereochemical outcomes:
- Frontier Molecular Orbital (FMO) analysis : Identifies electron-rich (HOMO) and electron-poor (LUMO) regions, guiding predictions of electrophilic or nucleophilic attack .
- Transition state modeling : Simulates activation energies for syn vs. anti addition pathways.
Validation : Compare computational results with experimental kinetic data (e.g., reaction rates under varying temperatures) .
Q. Advanced: How do steric effects in this compound influence its catalytic hydrogenation kinetics?
Answer:
The cis configuration creates steric hindrance near the double bond, slowing hydrogenation rates. Methodological approaches include:
- Kinetic studies : Monitor H2 uptake under controlled pressures and temperatures using manometric techniques.
- Catalyst optimization : Test palladium, platinum, or nickel catalysts on supports (e.g., carbon, alumina) to assess steric accessibility .
- Isotopic labeling : Use deuterium (D2) to track regioselectivity and confirm steric vs. electronic influences .
Data contradiction : Conflicting reports on reaction rates may arise from solvent effects (polar vs. nonpolar) or catalyst dispersion. Replicate experiments with standardized conditions.
Q. Advanced: What analytical strategies resolve discrepancies in reported thermodynamic properties (e.g., boiling points) of this compound?
Answer:
Discrepancies in properties like boiling points (~110–120°C) may stem from isomer impurities or measurement techniques:
- Differential Scanning Calorimetry (DSC) : Accurately measures enthalpy of vaporization and phase transitions .
- High-resolution GC-MS : Detects trace impurities (e.g., trans isomers or alkyl halide byproducts) that skew boiling point data .
- Meta-analysis : Cross-reference literature using databases like SciFinder or Reaxys to identify outliers and experimental conditions .
Best practice : Report purity levels (≥98% by GC) and calibration standards in publications.
Q. Advanced: How can reaction mechanisms involving this compound be validated using isotopic labeling or kinetic isotope effects (KIE)?
Answer:
To confirm mechanistic pathways (e.g., radical vs. ionic intermediates):
- Deuterium labeling : Introduce D at the double bond; observe KIE in reaction rates (e.g., C-D vs. C-H bond cleavage) .
- <sup>13</sup>C NMR tracking : Monitor carbon environments during reactions to identify intermediates.
- Computational validation : Compare experimental KIEs with DFT-derived activation barriers for proposed mechanisms .
Q. Basic: What are the best practices for safely handling and storing this compound in laboratory settings?
Answer:
- Storage : Under inert gas (N2 or Ar) at –20°C to prevent oxidation or polymerization .
- Handling : Use sealed Schlenk lines or gloveboxes to minimize air exposure.
- Decomposition monitoring : Regular GC analysis to detect degradation products (e.g., peroxides).
Propiedades
IUPAC Name |
(Z)-2-methylhex-3-ene | |
---|---|---|
Source | PubChem | |
URL | https://pubchem.ncbi.nlm.nih.gov | |
Description | Data deposited in or computed by PubChem | |
InChI |
InChI=1S/C7H14/c1-4-5-6-7(2)3/h5-7H,4H2,1-3H3/b6-5- | |
Source | PubChem | |
URL | https://pubchem.ncbi.nlm.nih.gov | |
Description | Data deposited in or computed by PubChem | |
InChI Key |
IQANHWBWTVLDTP-WAYWQWQTSA-N | |
Source | PubChem | |
URL | https://pubchem.ncbi.nlm.nih.gov | |
Description | Data deposited in or computed by PubChem | |
Canonical SMILES |
CCC=CC(C)C | |
Source | PubChem | |
URL | https://pubchem.ncbi.nlm.nih.gov | |
Description | Data deposited in or computed by PubChem | |
Isomeric SMILES |
CC/C=C\C(C)C | |
Source | PubChem | |
URL | https://pubchem.ncbi.nlm.nih.gov | |
Description | Data deposited in or computed by PubChem | |
Molecular Formula |
C7H14 | |
Source | PubChem | |
URL | https://pubchem.ncbi.nlm.nih.gov | |
Description | Data deposited in or computed by PubChem | |
DSSTOX Substance ID |
DTXSID301015952 | |
Record name | (3Z)-2-Methylhex-3-ene | |
Source | EPA DSSTox | |
URL | https://comptox.epa.gov/dashboard/DTXSID301015952 | |
Description | DSSTox provides a high quality public chemistry resource for supporting improved predictive toxicology. | |
Molecular Weight |
98.19 g/mol | |
Source | PubChem | |
URL | https://pubchem.ncbi.nlm.nih.gov | |
Description | Data deposited in or computed by PubChem | |
CAS No. |
15840-60-5 | |
Record name | 3-Hexene, 2-methyl-, (Z)- | |
Source | ChemIDplus | |
URL | https://pubchem.ncbi.nlm.nih.gov/substance/?source=chemidplus&sourceid=0015840605 | |
Description | ChemIDplus is a free, web search system that provides access to the structure and nomenclature authority files used for the identification of chemical substances cited in National Library of Medicine (NLM) databases, including the TOXNET system. | |
Record name | (3Z)-2-Methylhex-3-ene | |
Source | EPA DSSTox | |
URL | https://comptox.epa.gov/dashboard/DTXSID301015952 | |
Description | DSSTox provides a high quality public chemistry resource for supporting improved predictive toxicology. | |
Retrosynthesis Analysis
AI-Powered Synthesis Planning: Our tool employs the Template_relevance Pistachio, Template_relevance Bkms_metabolic, Template_relevance Pistachio_ringbreaker, Template_relevance Reaxys, Template_relevance Reaxys_biocatalysis model, leveraging a vast database of chemical reactions to predict feasible synthetic routes.
One-Step Synthesis Focus: Specifically designed for one-step synthesis, it provides concise and direct routes for your target compounds, streamlining the synthesis process.
Accurate Predictions: Utilizing the extensive PISTACHIO, BKMS_METABOLIC, PISTACHIO_RINGBREAKER, REAXYS, REAXYS_BIOCATALYSIS database, our tool offers high-accuracy predictions, reflecting the latest in chemical research and data.
Strategy Settings
Precursor scoring | Relevance Heuristic |
---|---|
Min. plausibility | 0.01 |
Model | Template_relevance |
Template Set | Pistachio/Bkms_metabolic/Pistachio_ringbreaker/Reaxys/Reaxys_biocatalysis |
Top-N result to add to graph | 6 |
Feasible Synthetic Routes
Descargo de responsabilidad e información sobre productos de investigación in vitro
Tenga en cuenta que todos los artículos e información de productos presentados en BenchChem están destinados únicamente con fines informativos. Los productos disponibles para la compra en BenchChem están diseñados específicamente para estudios in vitro, que se realizan fuera de organismos vivos. Los estudios in vitro, derivados del término latino "in vidrio", involucran experimentos realizados en entornos de laboratorio controlados utilizando células o tejidos. Es importante tener en cuenta que estos productos no se clasifican como medicamentos y no han recibido la aprobación de la FDA para la prevención, tratamiento o cura de ninguna condición médica, dolencia o enfermedad. Debemos enfatizar que cualquier forma de introducción corporal de estos productos en humanos o animales está estrictamente prohibida por ley. Es esencial adherirse a estas pautas para garantizar el cumplimiento de los estándares legales y éticos en la investigación y experimentación.