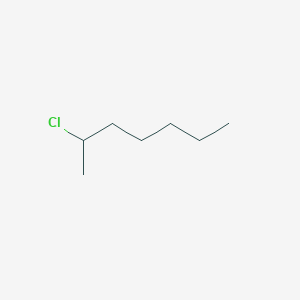
2-Chloroheptane
Descripción general
Descripción
2-Chloroheptane (C₇H₁₅Cl) is a secondary alkyl halide with a molecular weight of 134.647 g/mol and CAS number 1001-89-4 . Its structure features a chlorine atom at the second carbon of a seven-carbon chain, making it a versatile model compound for studying elimination, substitution, and catalytic dehydrochlorination reactions. Key identifiers include the ChemSpider ID 31926 and EINECS 213-683-7 .
Métodos De Preparación
Radical Chlorination of Heptane
Radical chlorination represents the most direct method for synthesizing 2-chloroheptane from heptane. This process leverages a free-radical chain mechanism initiated by ultraviolet (UV) light or radical initiators such as benzoyl peroxide.
Reaction Mechanism and Conditions
The chlorination proceeds via three stages: initiation, propagation, and termination. Initiation involves homolytic cleavage of chlorine (Cl₂) into chlorine radicals under UV light. Propagation steps include hydrogen abstraction from heptane by chlorine radicals, forming heptyl radicals, which subsequently react with Cl₂ to yield chloroheptane and regenerate chlorine radicals.
Key parameters influencing regioselectivity and yield include:
-
Temperature : Moderate temperatures (25–50°C) favor secondary chlorination due to the stability of secondary radicals.
-
Cl₂ Concentration : Excess chlorine gas increases reaction rate but may lead to over-chlorination.
-
Solvent : Non-polar solvents like carbon tetrachloride (CCl₄) enhance solubility of heptane and Cl₂.
Byproduct Formation and Selectivity
Radical chlorination inherently produces a mixture of structural isomers. For heptane, the secondary C-2 position is statistically favored, but tertiary positions (C-3 and C-4) may also form due to radical stability. Typical isomer distributions under optimized conditions are:
Isomer | Percentage Yield |
---|---|
This compound | 45–55% |
3-Chloroheptane | 25–30% |
1-Chloroheptane | 10–15% |
Separation techniques such as fractional distillation or chromatography are required to isolate this compound.
Hydrochlorination of 1-Heptene
Hydrochlorination of 1-heptene offers a more regioselective route to this compound through acid-catalyzed addition of hydrogen chloride (HCl).
Acid-Catalyzed Mechanism
The reaction follows Markovnikov’s rule, where HCl adds across the double bond to form the more stable secondary carbocation intermediate. Subsequent chloride ion attack yields this compound as the major product.
Conditions :
-
Catalyst : Lewis acids like zinc chloride (ZnCl₂) or aluminum chloride (AlCl₃) enhance reaction rate.
-
Temperature : 0–25°C to minimize carbocation rearrangements.
-
Solvent : Dichloromethane or ether improves reagent miscibility.
Yield Optimization
Under ideal conditions, 1-heptene conversion exceeds 90%, with this compound selectivity reaching 80–85%. Side products include 1-chloroheptane (5–10%) and dimerization byproducts from carbocation rearrangements.
Alcohol Substitution with Thionyl Chloride (SOCl₂)
Substitution of 2-heptanol with thionyl chloride is a high-yield, laboratory-friendly method. The reaction proceeds via a two-step mechanism:
-
Formation of Chlorosulfite Intermediate : 2-Heptanol reacts with SOCl₂ to generate an intermediate and HCl gas.
-
Nucleophilic Displacement : The chlorosulfite intermediate undergoes intramolecular displacement, releasing SO₂ and yielding this compound.
Advantages :
-
Purity : Minimal byproducts due to gaseous SO₂ and HCl evolution.
-
Conditions : Reactions proceed at room temperature with stoichiometric SOCl₂.
-
Yield : Typically 85–95% after distillation.
Comparative Analysis of Preparation Methods
Table 1: Method Efficiency and Scalability
Method | Yield (%) | Selectivity (%) | Scalability | Cost Efficiency |
---|---|---|---|---|
Radical Chlorination | 45–55 | 50–60 | Industrial | Low |
Hydrochlorination | 80–85 | 80–85 | Laboratory | Moderate |
SOCl₂ Substitution | 85–95 | >95 | Laboratory | High |
Key Considerations:
-
Radical Chlorination : Preferred for bulk production despite lower selectivity due to low-cost heptane feedstock.
-
Hydrochlorination : Balances yield and selectivity but requires pure alkene starting material.
-
SOCl₂ Substitution : Ideal for small-scale, high-purity synthesis but limited by SOCl₂ toxicity.
Industrial-Scale Production Challenges
Industrial synthesis of this compound prioritizes cost-effectiveness and safety. Radical chlorination dominates due to:
-
Feedstock Availability : Heptane is a petroleum derivative with established supply chains.
-
Continuous Reactor Design : Tubular reactors with UV lamps optimize Cl₂ utilization and minimize overheating.
Environmental and Safety Concerns :
-
Chlorine gas handling requires stringent safety protocols to prevent leaks.
-
Byproduct HCl must be neutralized or recycled to mitigate environmental impact.
Emerging Methodologies and Innovations
Recent advances focus on catalytic systems to enhance selectivity and reduce energy consumption:
Photoredox Catalysis
Visible-light-driven catalysis using ruthenium or iridium complexes enables precise control over radical formation, improving this compound selectivity to 70–75% at ambient temperatures.
Enzymatic Chlorination
Engineered halohydrin dehalogenases show promise in catalyzing regioselective chlorination under mild conditions, though scalability remains a challenge.
Análisis De Reacciones Químicas
2-Chloroheptane undergoes various chemical reactions, including:
Substitution Reactions:
Nucleophilic Substitution: this compound can undergo nucleophilic substitution reactions where the chlorine atom is replaced by a nucleophile. Common reagents include sodium hydroxide (NaOH) or potassium cyanide (KCN).
Elimination Reactions:
Dehydrohalogenation: In the presence of a strong base like potassium tert-butoxide, this compound can undergo elimination to form heptenes.
Oxidation Reactions:
Oxidation: Although less common, this compound can be oxidized to form corresponding alcohols or ketones under specific conditions.
Aplicaciones Científicas De Investigación
Overview
2-Chloroheptane (C7H15Cl) is a chlorinated alkane that serves as a versatile compound in various scientific and industrial applications. It is characterized by its molecular weight of approximately 134.65 g/mol and is commonly utilized as an intermediate in organic synthesis, a reagent in chemical reactions, and in biochemical studies. This article explores its applications across different fields, supported by data tables and case studies.
Chemical Synthesis
Intermediate in Organic Synthesis:
this compound is primarily used as an intermediate in the synthesis of various organic compounds. Its structure allows for nucleophilic substitution reactions, where the chlorine atom can be replaced by other functional groups. This property makes it valuable in creating more complex molecules necessary for pharmaceuticals and agrochemicals.
Reagent in Chemical Reactions:
In addition to serving as an intermediate, this compound is employed in studying reaction mechanisms and kinetics. Its reactivity as an alkyl halide facilitates investigations into nucleophilic substitution and elimination reactions, providing insights into fundamental organic chemistry principles.
Biochemical Studies
Impact on Biological Systems:
Research has shown that this compound can influence biological membranes due to its lipophilic nature. Studies have focused on its interactions with cellular components, which may elucidate the effects of chlorinated hydrocarbons on living organisms. Such investigations are crucial for understanding environmental toxicity and the biological implications of exposure to chlorinated compounds.
Industrial Applications
Solvent Properties:
Due to its nonpolar characteristics, this compound is occasionally utilized as a solvent in industrial applications. Its ability to dissolve various nonpolar substances makes it suitable for processes requiring specific solvent properties.
Chemical Manufacturing:
this compound plays a role in the production of other chemicals and materials, particularly in the synthesis of polymers like polyvinyl chloride (PVC). It acts as a model compound for studying catalytic systems used in polymerization processes.
Case Studies
-
Vibrational Analysis:
A study conducted on the vibrational frequencies of this compound utilized infrared spectroscopy to identify fundamental vibrations, aiding in understanding its molecular structure and behavior under various conditions . -
Catalytic Conversion:
Research examining the influence of catalytic systems on the conversion of this compound highlighted its role as a model compound for PVC production. The study detailed various side reactions that occur during its conversion, providing insights into optimizing industrial processes . -
Biological Interaction Studies:
Investigations into how this compound interacts with biological membranes revealed its potential toxicity profile and environmental behavior, emphasizing the need for further studies on chlorinated hydrocarbons' effects on living systems .
Mecanismo De Acción
The mechanism of action of 2-Chloroheptane primarily involves its reactivity as an alkyl halide. The chlorine atom in this compound is a good leaving group, making it susceptible to nucleophilic substitution and elimination reactions. The molecular targets and pathways involved include:
Nucleophilic Attack: The chlorine atom is replaced by a nucleophile, forming a new bond with the carbon atom.
Elimination: The removal of hydrogen and chlorine atoms leads to the formation of double bonds in the carbon chain.
Comparación Con Compuestos Similares
Structural and Reactivity Differences
Primary vs. Secondary Chlorides
- 1-Chloroheptane (primary chloride): The chlorine atom at the terminal carbon leads to faster SN2 reactivity due to reduced steric hindrance. However, in SN1 reactions , its primary carbocation is less stable than the secondary carbocation of 2-chloroheptane, resulting in slower SN1 rates .
- This compound (secondary chloride): Exhibits faster SN1 reactivity due to the stability of the secondary carbocation. For example, in SN1 reactions, this compound reacts faster than 1-chlorohexane (primary) but slower than tertiary chlorides like 2-chloro-2-methylpropane .
Branching and Catalytic Behavior
- 3-Chlorohexane : Branching at the third carbon reduces enzymatic activity. In studies with the LinB enzyme, 3-chlorohexane showed a reaction rate of 30 (UT26 strain), significantly lower than this compound (65) and 1-chloroheptane (96) .
- ZnCl₂/Ru-Catalyzed Reactions : Under catalytic dehydrochlorination (140°C, H₂), this compound undergoes HCl elimination to form heptene (67% conversion with ZnCl₂ alone) and further hydrogenation to heptane (with Ru). By contrast, 1-chloroheptane primarily forms linear alkenes without significant branching .
Reaction Pathways and Byproduct Formation
Elimination vs. Substitution
- This compound : Dominant elimination pathway under basic conditions (e.g., E2 mechanism) due to steric hindrance at the β-hydrogen. In contrast, 1-chloroheptane favors substitution (SN2) .
- Mass Spectrometry : this compound shows an intense peak at m/z 98 (loss of HCl), while 1-bromohexane exhibits weak HBr elimination (m/z 84). This highlights the influence of halide type and position on fragmentation patterns .
Carbocation Rearrangements
In ZnCl₂-catalyzed reactions, this compound generates carbocations prone to rearrangements, yielding branched products like methylhexane (7–10% yield) and chlorinated derivatives. Comparatively, 1-chloroheptane produces fewer branched byproducts due to less stable primary carbocations .
Enzymatic and Environmental Interactions
LinB Enzyme Activity
- Substrate Specificity : LinB enzyme from Sphingomonas paucimobilis shows higher activity toward 1-chloroheptane (reaction rate: 96 for UT26 strain) than this compound (65). The enzyme’s active site accommodates linear substrates more efficiently .
- Environmental Degradation : The secondary chloride in this compound slows biodegradation compared to primary chlorides, increasing persistence in ecosystems .
Spectroscopic Properties
- IR/Raman Spectra : this compound’s vibrational modes were analyzed using force constants from 2-chlorohexane studies. Differences in chain length alter peak positions, with observed frequencies assigned to five conformers (±6.4 cm⁻¹ error) .
- NMR Characterization : In dehydrochlorination studies, ¹H-NMR detected residual CHCl groups (δ 4.7–4.3 ppm) and branching indicators (δ 0.9 ppm for CH₃ groups) .
Data Tables
Table 1: Molecular Properties of Selected Chloroalkanes
Compound | Molecular Formula | Molecular Weight | Chlorine Position | Reactivity (SN1) | Reactivity (SN2) |
---|---|---|---|---|---|
This compound | C₇H₁₅Cl | 134.647 | Secondary | High | Moderate |
1-Chloroheptane | C₇H₁₅Cl | 134.647 | Primary | Low | High |
3-Chlorohexane | C₆H₁₃Cl | 120.620 | Secondary | Moderate | Low |
Table 2: Catalytic Dehydrochlorination of this compound
Catalyst | Conversion (%) | Main Product | Branched Byproducts (%) |
---|---|---|---|
ZnCl₂ | 67 | Heptene | 7 |
ZnCl₂/Ru | 58 | Heptane | 10 |
None (Control) | 0 | — | — |
Actividad Biológica
Overview
2-Chloroheptane, an organic compound with the molecular formula CHCl, is a chlorinated derivative of heptane. This compound is primarily utilized in organic synthesis and as a reagent in chemical reactions. Its biological activity has garnered attention due to its potential effects on living organisms and its applications in biochemical studies.
- Molecular Formula : CHCl
- Molecular Weight : 134.65 g/mol
- Boiling Point : 146 °C
- Density : 0.881 g/mL at 25 °C
- Appearance : Colorless to almost colorless liquid
Biological Activity
The biological activity of this compound can be categorized into several key areas:
1. Toxicological Effects
Research indicates that chlorinated hydrocarbons, including this compound, may exhibit toxic effects on various biological systems. Studies have shown that exposure to chlorinated compounds can lead to adverse effects on human health and the environment. For instance, chlorinated aliphatic hydrocarbons are known to disrupt endocrine functions and may have carcinogenic properties.
2. Microbial Dehalogenation
A significant area of study involves the microbial degradation of haloalkanes, including this compound. Certain strains of bacteria, such as Mycobacterium tuberculosis, have demonstrated dehalogenation capabilities, converting haloalkanes into less harmful alcohols through hydrolytic mechanisms. This process is critical for bioremediation efforts aimed at detoxifying environments contaminated with chlorinated compounds .
3. Biochemical Studies
This compound has been employed in biochemical studies to understand the interactions between chlorinated hydrocarbons and biological systems. These studies often focus on the compound's reactivity and its potential as a substrate for enzymatic reactions. Research has indicated that the secondary carbon-chlorine bond in this compound is more reactive than primary bonds found in other chlorinated hydrocarbons, making it a valuable subject for investigating nucleophilic substitution reactions .
Case Study 1: Toxicity Assessment
A toxicity assessment was conducted using various concentrations of this compound on cultured human cells. The results indicated that higher concentrations led to significant cytotoxicity, characterized by reduced cell viability and increased apoptosis rates. This study highlights the need for careful handling and regulation of chlorinated compounds in industrial applications.
Concentration (µM) | Cell Viability (%) | Apoptosis Rate (%) |
---|---|---|
0 | 100 | 5 |
10 | 85 | 10 |
50 | 60 | 25 |
100 | 30 | 50 |
Case Study 2: Microbial Degradation
In a study examining the microbial degradation of haloalkanes, researchers isolated Mycobacterium strains capable of degrading various chlorinated compounds, including this compound. The study found that these bacteria could effectively convert the compound into its corresponding alcohol, demonstrating their potential use in bioremediation.
Bacterial Strain | Dehalogenation Efficiency (%) |
---|---|
Mycobacterium tuberculosis H37Rv | 75 |
Mycobacterium smegmatis | 65 |
Mycobacterium bovis | 50 |
Q & A
Basic Research Questions
Q. How can spectroscopic methods confirm the molecular structure of 2-Chloroheptane?
- Methodological Answer :
- Mass Spectrometry (MS) : Analyze fragmentation patterns. The molecular ion peak at m/z 134 ([M]⁺) corresponds to C₇H₁₅Cl. Key fragments include m/z 98 (loss of HCl) and m/z 57 (C₄H₉⁺), consistent with alkyl chloride fragmentation .
- NMR Spectroscopy : Use ¹H-NMR to identify protons adjacent to the chlorine atom. Peaks at δ 4.7–4.3 ppm (CHCl) and δ 1.28–2.0 ppm (CH₂ groups) confirm the secondary chloride structure. Integration ratios validate proton distribution .
- Infrared (IR) : Absorbance near 550–650 cm⁻¹ (C-Cl stretch) supports halogen presence .
Q. What safety protocols are critical when handling this compound in laboratory settings?
- Methodological Answer :
- Ventilation : Use fume hoods to avoid inhalation of vapors.
- Personal Protective Equipment (PPE) : Wear nitrile gloves (0.7 mm thickness, >480 min penetration resistance) and chemical-resistant aprons. Eye protection must meet EN 166 or NIOSH standards .
- Storage : Keep in airtight containers under inert gas (e.g., N₂) to prevent moisture exposure .
Q. How is the purity of this compound determined experimentally?
- Methodological Answer :
- Gas Chromatography (GC) : Use an internal standard (e.g., decane) and Flame Ionization Detection (FID). Calculate purity via effective carbon number (ECN) correction factors (ECN = 6.88 for this compound) .
- ¹H-NMR Integration : Compare the relative integrals of CHCl (δ 4.7–4.3 ppm) and CH₃ (δ 0.9 ppm) groups. Normalize to 100% to quantify residual impurities .
Advanced Research Questions
Q. How do catalytic systems influence the dehydrochlorination efficiency of this compound?
- Methodological Answer :
- Catalyst Screening : Test ZnCl₂ and HCl(CO)Ru(PPh₃)₃ under H₂ (20 bar) at 140°C. Monitor conversion via GC-MS, noting higher selectivity for heptane with Ru catalysts due to enhanced β-H elimination pathways .
- XAS Analysis : Use Ru K-edge XANES to study electronic changes in the catalyst. Compare fresh vs. spent catalysts to identify Ru oxidation state shifts during reaction cycles .
Q. How can contradictions in SN1/SN2 reaction kinetics for this compound be resolved?
- Methodological Answer :
- Solvent Effects : Polar protic solvents (e.g., ethanol) favor SN1 (via carbocation stabilization: 2° carbocation from this compound). Polar aprotic solvents (e.g., DMSO) favor SN2 (steric hindrance limits backside attack) .
- Kinetic Isotope Studies : Replace Cl with ³⁷Cl to track isotopic scrambling in SN1 pathways. Use ²H-labeled substrates to distinguish between concerted (SN2) and stepwise (SN1) mechanisms .
Q. What advanced techniques characterize residual chlorine in polymer products derived from this compound?
- Methodological Answer :
- ¹H-NMR Quantification : Integrate CHCl peaks (δ 4.7–4.3 ppm) and normalize to theoretical proton counts. Calculate dechlorination efficiency as .
- GC-MS with Isotopic Labeling : Use ²H- or ¹³C-labeled this compound to trace chlorine redistribution during copolymerization .
Q. Data Contradiction Analysis
Q. How to address discrepancies in reported catalytic efficiencies for this compound dehydrochlorination?
- Methodological Answer :
- Standardize Reaction Conditions : Compare studies using identical parameters (140°C, 20 bar H₂, 16 h). Note deviations in catalyst loading or H₂ purity.
- Cross-Validate with XAS : Confirm active Ru species (e.g., Ru⁰ vs. Ru²⁺) across studies. Inconsistent pretreatment (e.g., reduction under H₂) may explain efficiency gaps .
Q. Why do mass spectral fragmentation patterns of this compound vary across instruments?
- Methodological Answer :
- Ionization Energy Calibration : Adjust electron ionization (EI) energy (70 eV standard) to minimize instrument-specific fragmentation.
- Reference Standardization : Compare peaks to NIST database entries and decane internal standards for retention time alignment .
Propiedades
IUPAC Name |
2-chloroheptane | |
---|---|---|
Source | PubChem | |
URL | https://pubchem.ncbi.nlm.nih.gov | |
Description | Data deposited in or computed by PubChem | |
InChI |
InChI=1S/C7H15Cl/c1-3-4-5-6-7(2)8/h7H,3-6H2,1-2H3 | |
Source | PubChem | |
URL | https://pubchem.ncbi.nlm.nih.gov | |
Description | Data deposited in or computed by PubChem | |
InChI Key |
PTSLUOSUHFGQHV-UHFFFAOYSA-N | |
Source | PubChem | |
URL | https://pubchem.ncbi.nlm.nih.gov | |
Description | Data deposited in or computed by PubChem | |
Canonical SMILES |
CCCCCC(C)Cl | |
Source | PubChem | |
URL | https://pubchem.ncbi.nlm.nih.gov | |
Description | Data deposited in or computed by PubChem | |
Molecular Formula |
C7H15Cl | |
Source | PubChem | |
URL | https://pubchem.ncbi.nlm.nih.gov | |
Description | Data deposited in or computed by PubChem | |
DSSTOX Substance ID |
DTXSID80905206 | |
Record name | 2-Chloroheptane | |
Source | EPA DSSTox | |
URL | https://comptox.epa.gov/dashboard/DTXSID80905206 | |
Description | DSSTox provides a high quality public chemistry resource for supporting improved predictive toxicology. | |
Molecular Weight |
134.65 g/mol | |
Source | PubChem | |
URL | https://pubchem.ncbi.nlm.nih.gov | |
Description | Data deposited in or computed by PubChem | |
CAS No. |
1001-89-4 | |
Record name | 2-Chloroheptane | |
Source | CAS Common Chemistry | |
URL | https://commonchemistry.cas.org/detail?cas_rn=1001-89-4 | |
Description | CAS Common Chemistry is an open community resource for accessing chemical information. Nearly 500,000 chemical substances from CAS REGISTRY cover areas of community interest, including common and frequently regulated chemicals, and those relevant to high school and undergraduate chemistry classes. This chemical information, curated by our expert scientists, is provided in alignment with our mission as a division of the American Chemical Society. | |
Explanation | The data from CAS Common Chemistry is provided under a CC-BY-NC 4.0 license, unless otherwise stated. | |
Record name | 2-Chloroheptane | |
Source | ChemIDplus | |
URL | https://pubchem.ncbi.nlm.nih.gov/substance/?source=chemidplus&sourceid=0001001894 | |
Description | ChemIDplus is a free, web search system that provides access to the structure and nomenclature authority files used for the identification of chemical substances cited in National Library of Medicine (NLM) databases, including the TOXNET system. | |
Record name | 2-Chloroheptane | |
Source | EPA DSSTox | |
URL | https://comptox.epa.gov/dashboard/DTXSID80905206 | |
Description | DSSTox provides a high quality public chemistry resource for supporting improved predictive toxicology. | |
Record name | 2-chloroheptane | |
Source | European Chemicals Agency (ECHA) | |
URL | https://echa.europa.eu/substance-information/-/substanceinfo/100.012.439 | |
Description | The European Chemicals Agency (ECHA) is an agency of the European Union which is the driving force among regulatory authorities in implementing the EU's groundbreaking chemicals legislation for the benefit of human health and the environment as well as for innovation and competitiveness. | |
Explanation | Use of the information, documents and data from the ECHA website is subject to the terms and conditions of this Legal Notice, and subject to other binding limitations provided for under applicable law, the information, documents and data made available on the ECHA website may be reproduced, distributed and/or used, totally or in part, for non-commercial purposes provided that ECHA is acknowledged as the source: "Source: European Chemicals Agency, http://echa.europa.eu/". Such acknowledgement must be included in each copy of the material. ECHA permits and encourages organisations and individuals to create links to the ECHA website under the following cumulative conditions: Links can only be made to webpages that provide a link to the Legal Notice page. | |
Retrosynthesis Analysis
AI-Powered Synthesis Planning: Our tool employs the Template_relevance Pistachio, Template_relevance Bkms_metabolic, Template_relevance Pistachio_ringbreaker, Template_relevance Reaxys, Template_relevance Reaxys_biocatalysis model, leveraging a vast database of chemical reactions to predict feasible synthetic routes.
One-Step Synthesis Focus: Specifically designed for one-step synthesis, it provides concise and direct routes for your target compounds, streamlining the synthesis process.
Accurate Predictions: Utilizing the extensive PISTACHIO, BKMS_METABOLIC, PISTACHIO_RINGBREAKER, REAXYS, REAXYS_BIOCATALYSIS database, our tool offers high-accuracy predictions, reflecting the latest in chemical research and data.
Strategy Settings
Precursor scoring | Relevance Heuristic |
---|---|
Min. plausibility | 0.01 |
Model | Template_relevance |
Template Set | Pistachio/Bkms_metabolic/Pistachio_ringbreaker/Reaxys/Reaxys_biocatalysis |
Top-N result to add to graph | 6 |
Feasible Synthetic Routes
Descargo de responsabilidad e información sobre productos de investigación in vitro
Tenga en cuenta que todos los artículos e información de productos presentados en BenchChem están destinados únicamente con fines informativos. Los productos disponibles para la compra en BenchChem están diseñados específicamente para estudios in vitro, que se realizan fuera de organismos vivos. Los estudios in vitro, derivados del término latino "in vidrio", involucran experimentos realizados en entornos de laboratorio controlados utilizando células o tejidos. Es importante tener en cuenta que estos productos no se clasifican como medicamentos y no han recibido la aprobación de la FDA para la prevención, tratamiento o cura de ninguna condición médica, dolencia o enfermedad. Debemos enfatizar que cualquier forma de introducción corporal de estos productos en humanos o animales está estrictamente prohibida por ley. Es esencial adherirse a estas pautas para garantizar el cumplimiento de los estándares legales y éticos en la investigación y experimentación.