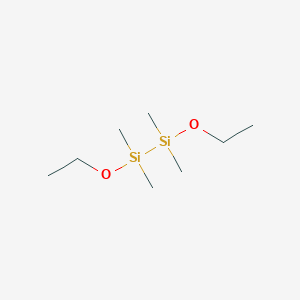
1,2-Dietoxi-1,1,2,2-tetrametil disilano
Descripción general
Descripción
A4I, también conocido como Análisis para Innovadores, es un compuesto que ha ganado una atención significativa en los últimos años debido a sus propiedades únicas y aplicaciones potenciales. Este compuesto se utiliza principalmente en varios campos de investigación científica, incluyendo química, biología, medicina e industria. Su estructura única y reactividad lo convierten en una herramienta valiosa para investigadores y científicos.
Aplicaciones Científicas De Investigación
Organic Synthesis
DEDTMS serves as a precursor in various organic synthesis reactions. One notable application is in the palladium-catalyzed bis(silylation) of compounds such as 1,4-diethynylbenzene. This reaction results in the formation of a crack-free sol-gel material, which has implications in materials science for creating durable coatings and films .
Silylation Reactions
Silylation is a critical process in organic chemistry that involves the introduction of silicon-containing groups into organic molecules. DEDTMS is particularly effective in modifying compounds with carbon-carbon double bonds. This modification can enhance the stability and reactivity of target molecules, making them suitable for further chemical transformations .
Preparation of Dimethylsilylene
DEDTMS can be thermally decomposed via gas-phase flow-pyrolysis at elevated temperatures (around 600 °C) to yield dimethylsilylene, a reactive species utilized in various synthetic pathways . This process highlights DEDTMS's role as a valuable intermediate in generating reactive silanes.
Case Study 1: Silylation of Unsaturated Compounds
A study demonstrated the effectiveness of DEDTMS in the silylation of various unsaturated organic compounds. The resulting silylated products exhibited enhanced thermal stability and reactivity compared to their non-silylated counterparts. This finding underscores DEDTMS's utility in synthesizing advanced materials with tailored properties .
Case Study 2: Sol-Gel Process Development
In another research project focused on sol-gel processes for coating applications, DEDTMS was employed to synthesize hybrid organic-inorganic materials. The resulting sol-gel exhibited excellent mechanical properties and thermal stability, making it suitable for protective coatings in various industrial applications .
Métodos De Preparación
Rutas Sintéticas y Condiciones de Reacción
La preparación de A4I implica varias rutas sintéticas y condiciones de reacción. Un método común es la oxidación de alcoholes primarios a aldehídos, seguida de una posterior oxidación para formar el compuesto deseado. Este proceso típicamente involucra el uso de reactivos inorgánicos como el trióxido de cromo y el permanganato de potasio . Otro método implica la reducción de ácidos carboxílicos a aldehídos, que luego pueden procesarse para obtener A4I .
Métodos de Producción Industrial
A escala industrial, A4I se puede producir a través de la reordenación de hidroperóxidos de alquilo. Este método implica la oxidación directa al aire de hidrocarburos o la adición de peróxido de hidrógeno a dobles enlaces, seguida de una reordenación inducida por ácido sulfúrico . Este proceso es eficiente y rentable, lo que lo hace adecuado para la producción a gran escala.
Análisis De Reacciones Químicas
Tipos de Reacciones
A4I experimenta varios tipos de reacciones químicas, incluyendo oxidación, reducción y sustitución. Estas reacciones son esenciales para su aplicación en diferentes campos.
Reactivos y Condiciones Comunes
Oxidación: Los reactivos comunes incluyen el trióxido de cromo y el permanganato de potasio. La reacción típicamente ocurre en condiciones ácidas.
Reducción: El borohidruro de sodio y el hidruro de aluminio y litio se utilizan comúnmente como agentes reductores. Estas reacciones generalmente tienen lugar en condiciones suaves para evitar la sobre-reducción.
Sustitución: Las reacciones de halogenación a menudo involucran reactivos como cloro o bromo, y la reacción ocurre en condiciones controladas de temperatura y presión.
Principales Productos Formados
Los principales productos formados a partir de estas reacciones incluyen varios aldehídos, cetonas y derivados sustituidos de A4I. Estos productos son valiosos intermediarios para la síntesis química y las aplicaciones posteriores.
Mecanismo De Acción
El mecanismo de acción de A4I implica su interacción con objetivos moleculares y vías específicas. Actúa principalmente modulando la actividad de ciertas enzimas y proteínas, lo que lleva a cambios en los procesos celulares. Los objetivos moleculares y las vías exactas pueden variar según la aplicación y el contexto específicos .
Comparación Con Compuestos Similares
A4I se puede comparar con otros compuestos similares, como aldehídos y cetonas. Si bien estos compuestos comparten algunas similitudes estructurales, A4I es único debido a su reactividad y aplicaciones específicas. Compuestos similares incluyen formaldehído, acetaldehído y benzaldehído .
Actividad Biológica
1,2-Diethoxy-1,1,2,2-tetramethyldisilane (DETDS) is an organosilicon compound that has garnered attention for its potential biological activities and applications in organic synthesis. This article explores the biological activity of DETDS, including its mechanisms of action, synthesis methods, and relevant case studies.
Chemical Structure and Properties
DETDS has the molecular formula . Its structure includes two ethoxy groups and a disilane backbone, which contributes to its unique reactivity profile. The presence of silicon atoms allows for interactions with various organic compounds through a process known as silylation.
The biological activity of DETDS primarily arises from its ability to undergo silylation reactions with organic compounds containing carbon-carbon double bonds. This process modifies the structure and properties of target molecules, potentially enhancing their utility in various applications. The following biochemical pathways are notably affected:
- Silylation Pathway : The addition of silicon atoms to organic compounds alters their physical and chemical properties.
- Volatility and Absorption : DETDS is volatile and can be absorbed through inhalation, which may have implications for its biological effects.
Synthesis Methods
DETDS can be synthesized through several methods, including:
- Palladium-Catalyzed Reactions : It is employed in palladium-catalyzed bis-silylation reactions to form complex silanes.
- Gas-Phase Flow-Pyrolysis : At high temperatures (around 600°C), DETDS can be converted into dimethylsilylene, a valuable precursor for various organosilicon compounds.
Biological Activity
Research indicates that DETDS exhibits biological activity primarily through its role in organic synthesis rather than direct pharmacological effects. The compound's interaction with other organic molecules can lead to the formation of new compounds with enhanced properties.
Case Studies
- Silylation Reactions : In a study involving the silylation of 1,4-diethynylbenzene using DETDS, researchers successfully synthesized crack-free sol-gels. This application highlights the compound's utility in material science.
- Reactivity with Functional Groups : DETDS has been shown to react with various functional groups in organic compounds. For instance, it was utilized in the preparation of tert-butyl 4-(hydroxydimethylsilyl)benzoate from tert-butyl 4-bromobenzoate via a palladium-catalyzed reaction .
Comparative Analysis
The following table compares DETDS with structurally similar compounds:
Compound Name | Molecular Formula | Key Features | Unique Aspects |
---|---|---|---|
1,2-Diethoxy-1,1,2,2-tetramethyldisilane | C8H18O4Si2 | Ethoxy groups | Different reactivity profile due to larger ethyl groups |
1,2-Difluoro-1,1,2,2-tetramethyldisilane | C6H12F2Si2 | Contains fluorine substituents | Higher electrophilicity due to electronegative fluorine |
1,3-Dimethyl-1-silacyclopentane | C6H14OSi | Cyclic structure with silicon | Distinct cyclic geometry affecting reactivity |
3-Methyl-3-silabicyclo[3.3.0]octane | C8H14Si | Bicyclic structure | Unique bicyclic framework influencing properties |
Propiedades
IUPAC Name |
ethoxy-[ethoxy(dimethyl)silyl]-dimethylsilane | |
---|---|---|
Source | PubChem | |
URL | https://pubchem.ncbi.nlm.nih.gov | |
Description | Data deposited in or computed by PubChem | |
InChI |
InChI=1S/C8H22O2Si2/c1-7-9-11(3,4)12(5,6)10-8-2/h7-8H2,1-6H3 | |
Source | PubChem | |
URL | https://pubchem.ncbi.nlm.nih.gov | |
Description | Data deposited in or computed by PubChem | |
InChI Key |
GWIVSKPSMYHUAK-UHFFFAOYSA-N | |
Source | PubChem | |
URL | https://pubchem.ncbi.nlm.nih.gov | |
Description | Data deposited in or computed by PubChem | |
Canonical SMILES |
CCO[Si](C)(C)[Si](C)(C)OCC | |
Source | PubChem | |
URL | https://pubchem.ncbi.nlm.nih.gov | |
Description | Data deposited in or computed by PubChem | |
Molecular Formula |
C8H22O2Si2 | |
Source | PubChem | |
URL | https://pubchem.ncbi.nlm.nih.gov | |
Description | Data deposited in or computed by PubChem | |
DSSTOX Substance ID |
DTXSID10460878 | |
Record name | 1,2-Diethoxy-1,1,2,2-tetramethyldisilane | |
Source | EPA DSSTox | |
URL | https://comptox.epa.gov/dashboard/DTXSID10460878 | |
Description | DSSTox provides a high quality public chemistry resource for supporting improved predictive toxicology. | |
Molecular Weight |
206.43 g/mol | |
Source | PubChem | |
URL | https://pubchem.ncbi.nlm.nih.gov | |
Description | Data deposited in or computed by PubChem | |
CAS No. |
18419-84-6 | |
Record name | 1,2-Diethoxy-1,1,2,2-tetramethyldisilane | |
Source | EPA DSSTox | |
URL | https://comptox.epa.gov/dashboard/DTXSID10460878 | |
Description | DSSTox provides a high quality public chemistry resource for supporting improved predictive toxicology. | |
Record name | 18419-84-6 | |
Source | European Chemicals Agency (ECHA) | |
URL | https://echa.europa.eu/information-on-chemicals | |
Description | The European Chemicals Agency (ECHA) is an agency of the European Union which is the driving force among regulatory authorities in implementing the EU's groundbreaking chemicals legislation for the benefit of human health and the environment as well as for innovation and competitiveness. | |
Explanation | Use of the information, documents and data from the ECHA website is subject to the terms and conditions of this Legal Notice, and subject to other binding limitations provided for under applicable law, the information, documents and data made available on the ECHA website may be reproduced, distributed and/or used, totally or in part, for non-commercial purposes provided that ECHA is acknowledged as the source: "Source: European Chemicals Agency, http://echa.europa.eu/". Such acknowledgement must be included in each copy of the material. ECHA permits and encourages organisations and individuals to create links to the ECHA website under the following cumulative conditions: Links can only be made to webpages that provide a link to the Legal Notice page. | |
Synthesis routes and methods
Procedure details
Retrosynthesis Analysis
AI-Powered Synthesis Planning: Our tool employs the Template_relevance Pistachio, Template_relevance Bkms_metabolic, Template_relevance Pistachio_ringbreaker, Template_relevance Reaxys, Template_relevance Reaxys_biocatalysis model, leveraging a vast database of chemical reactions to predict feasible synthetic routes.
One-Step Synthesis Focus: Specifically designed for one-step synthesis, it provides concise and direct routes for your target compounds, streamlining the synthesis process.
Accurate Predictions: Utilizing the extensive PISTACHIO, BKMS_METABOLIC, PISTACHIO_RINGBREAKER, REAXYS, REAXYS_BIOCATALYSIS database, our tool offers high-accuracy predictions, reflecting the latest in chemical research and data.
Strategy Settings
Precursor scoring | Relevance Heuristic |
---|---|
Min. plausibility | 0.01 |
Model | Template_relevance |
Template Set | Pistachio/Bkms_metabolic/Pistachio_ringbreaker/Reaxys/Reaxys_biocatalysis |
Top-N result to add to graph | 6 |
Feasible Synthetic Routes
Q1: How does 1,2-diethoxy-1,1,2,2-tetramethyldisilane interact with aryl bromides in the presence of a palladium catalyst, and what are the downstream effects of this interaction?
A1: In the presence of a palladium catalyst, 1,2-diethoxy-1,1,2,2-tetramethyldisilane inserts itself into the carbon-bromine bond of aryl bromides. [] This insertion leads to the formation of aryldimethylsilyl ethers. These ethers can then be readily hydrolyzed under controlled pH conditions to yield the desired aryldimethylsilanols. This palladium-catalyzed silylation offers a mild and efficient method for synthesizing aryldimethylsilanols, which are valuable building blocks in organic synthesis. []
Q2: Can you provide details about the structural characterization of 1,2-diethoxy-1,1,2,2-tetramethyldisilane?
A2: While the provided research abstract [] focuses on the application of 1,2-diethoxy-1,1,2,2-tetramethyldisilane, it does not delve into its detailed structural characterization. For comprehensive information on its molecular formula, weight, and spectroscopic data, it is recommended to consult resources like chemical databases (e.g., PubChem, ChemSpider) or the supplier's safety data sheet.
Descargo de responsabilidad e información sobre productos de investigación in vitro
Tenga en cuenta que todos los artículos e información de productos presentados en BenchChem están destinados únicamente con fines informativos. Los productos disponibles para la compra en BenchChem están diseñados específicamente para estudios in vitro, que se realizan fuera de organismos vivos. Los estudios in vitro, derivados del término latino "in vidrio", involucran experimentos realizados en entornos de laboratorio controlados utilizando células o tejidos. Es importante tener en cuenta que estos productos no se clasifican como medicamentos y no han recibido la aprobación de la FDA para la prevención, tratamiento o cura de ninguna condición médica, dolencia o enfermedad. Debemos enfatizar que cualquier forma de introducción corporal de estos productos en humanos o animales está estrictamente prohibida por ley. Es esencial adherirse a estas pautas para garantizar el cumplimiento de los estándares legales y éticos en la investigación y experimentación.