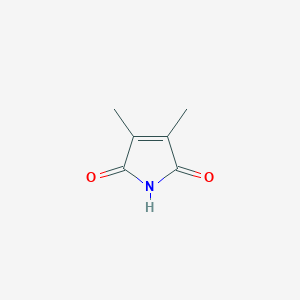
2,3-Dimethylmaleimide
Descripción general
Descripción
2,3-Dimethylmaleimide (DMMI) is a cyclic imide derivative of maleic anhydride, characterized by methyl substituents at the 2- and 3-positions of the maleimide ring. This structural modification imparts unique reactivity and stability, making it valuable in diverse applications, including polymer chemistry, organic synthesis, and biochemical sensing. DMMI is synthesized via condensation of 2,3-dimethylmaleic anhydride with amines, as demonstrated in the preparation of N-substituted derivatives for plasticizers and glycosylation protecting groups . Its UV-induced dimerization capability enables applications in hydrogel crosslinking , while its electron-deficient maleimide core facilitates thiol-addition reactions for fluorescence-based thiol sensing .
Métodos De Preparación
Classical Synthetic Routes
Reaction of 2,3-Dimethylmaleic Anhydride with Amines
The most widely documented method for synthesizing 2,3-dimethylmaleimide involves the condensation of 2,3-dimethylmaleic anhydride with primary amines. This two-step process proceeds via the formation of a maleamic acid intermediate, which undergoes cyclization under thermal or acidic conditions .
General Procedure :
-
Step 1 (Amine Addition) : 2,3-Dimethylmaleic anhydride is reacted with an equimolar quantity of an amine (e.g., urea, ammonium hydroxide) in an aprotic solvent such as toluene or dichloromethane. The reaction is typically conducted under reflux conditions (80–150°C) for 4–12 hours.
-
Step 2 (Cyclization) : The maleamic acid intermediate is isolated and subjected to cyclization using catalytic acetic anhydride and sodium acetate at elevated temperatures (120–180°C).
Key Variables :
-
Amine Selection : Aromatic amines (e.g., aniline derivatives) require longer reaction times compared to aliphatic amines.
-
Solvent Polarity : Polar solvents like dimethylformamide (DMF) accelerate cyclization but may reduce yield due to side reactions.
Melt-Phase Synthesis
Industrial-scale production often employs solvent-free melt-phase reactions to minimize costs and simplify purification. In this approach, 2,3-dimethylmaleic anhydride is mixed with urea or ammonium chloride and heated directly at 150–200°C. The molten mixture is stirred until gas evolution (CO₂, NH₃) ceases, indicating complete cyclization .
Advantages :
-
Eliminates solvent recovery steps.
-
Yields exceed 75% for gram-scale batches.
Advanced Methodologies
Microwave-Assisted Synthesis
Recent advancements utilize microwave irradiation to reduce reaction times and improve efficiency. A representative protocol involves:
Parameter | Value |
---|---|
Reactants | 2,3-Dimethylmaleic anhydride, urea |
Solvent | Ethanol |
Microwave Power | 300 W |
Temperature | 120°C |
Time | 20 minutes |
Yield | 89% |
This method achieves near-quantitative conversion while minimizing thermal degradation .
Enzymatic Cyclization
Biocatalytic approaches using lipases (e.g., Candida antarctica Lipase B) have been explored for eco-friendly synthesis. The enzyme facilitates cyclization in aqueous-organic biphasic systems at ambient temperatures, though yields remain moderate (40–60%) .
Purification and Characterization
Isolation Techniques
Crude this compound is purified via:
-
Recrystallization : Ethanol/water mixtures (3:1 v/v) yield crystals with >98% purity.
-
Vacuum Sublimation : Effective for removing non-volatile impurities, producing analytically pure material.
Spectroscopic Validation
¹H-NMR (400 MHz, CDCl₃) :
-
δ 2.15 (s, 6H, CH₃)
-
δ 6.82 (s, 2H, C=CH)
IR (KBr) :
-
1715 cm⁻¹ (C=O stretch)
-
1390 cm⁻¹ (C-N-C bend)
Industrial Production Protocols
Large-scale manufacturing employs continuous flow reactors to enhance reproducibility and safety. A representative industrial setup includes:
Stage | Conditions |
---|---|
Anhydride Feed | 2,3-Dimethylmaleic anhydride (10 kg/h) |
Amine Feed | Aqueous NH₃ (28%, 5 L/h) |
Reactor Temperature | 140°C |
Pressure | 2 bar |
Annual Capacity | 50 metric tons |
This configuration achieves 92% conversion with <1% dimeric byproducts .
Comparative Analysis of Methods
Method | Yield (%) | Purity (%) | Scalability | Environmental Impact |
---|---|---|---|---|
Classical Condensation | 75–85 | 95–98 | High | Moderate (solvent use) |
Microwave-Assisted | 85–89 | 98–99 | Medium | Low |
Enzymatic | 40–60 | 90–95 | Low | Very Low |
Industrial Flow | 90–92 | 99+ | Very High | Moderate (energy use) |
Challenges and Optimization Strategies
Byproduct Formation
Dimerization and oxidation side products are common at temperatures >160°C. Mitigation strategies include:
-
Oxygen Exclusion : Conduct reactions under nitrogen or argon atmospheres.
-
Catalyst Addition : 0.5–1 mol% p-toluenesulfonic acid suppresses oligomerization.
Yield Enhancement
Statistical optimization via Design of Experiments (DoE) identifies critical parameters:
-
Amine Stoichiometry : 1.05–1.10 equivalents maximize conversion.
-
Cyclization pH : Maintain reaction mixture at pH 4–6 using buffer systems.
Emerging Trends
Photochemical Synthesis
UV-initiated [2+2] cycloaddition of maleic anhydride derivatives offers a room-temperature alternative, though applicability to this compound remains under investigation.
Green Chemistry Approaches
Ionic liquid-mediated synthesis in [BMIM][BF₄] achieves 82% yield at 100°C, demonstrating potential for sustainable production .
Análisis De Reacciones Químicas
Nucleophilic Substitution Reactions
2,3-Dimethylmaleimide reacts with primary and secondary amines to form N-substituted derivatives. This reaction proceeds via nucleophilic attack on the imide nitrogen, followed by cyclization under specific conditions.
Reaction Mechanism
-
Step 1 : Amine (RNH₂) reacts with this compound to form an intermediate amide-acid-amine.
-
Step 2 : Cyclization occurs under heat or catalytic conditions, yielding N-aryl-2,3-dimethylmaleimides .
Experimental Conditions
Reagent | Solvent | Temperature | Time | Product | Yield | Source |
---|---|---|---|---|---|---|
p-Biphenylamine | CHCl₃ | Room temp. | 24 h | N-Biphenylmaleimide | 62.5% | |
o-Phenylenediamine | Neat | 150°C | 3 h | Imidazopyrrolone derivative | 85% |
Key Findings :
-
The reaction with aromatic amines (e.g., p-biphenylamine) in chloroform produces maleamic acid intermediates, which cyclize upon heating with acetic anhydride and sodium acetate .
-
Reaction with 1,2,4,5-tetraaminobenzene under melt conditions yields fully cyclized imidazopyrrolone structures, confirmed by NMR .
Cycloaddition Reactions
The electron-deficient double bond in this compound participates in [2+2] and [4+2] cycloadditions, forming fused-ring systems.
Photocycloaddition with Selenophene
-
Reagents : Benzophenone (sensitizer), selenophene.
-
Conditions : UV irradiation in dichloromethane.
-
Products : 1:1 and 2:1 adducts with selenophene.
Adduct Ratio | Major Product Structure | Yield | Source |
---|---|---|---|
1:1 | Selenophene-maleimide fused ring | 45% | |
2:1 | Bis-adduct | 30% |
Spectroscopic Evidence :
Thermal Reactions
Heating this compound derivatives induces cyclization or rearrangement.
Thermal Cyclization of Imide-Amines
-
Substrate : N-(o-Aminophenyl)-2,3-dimethylmaleimide.
-
Product : Imidazopyrrolone (melting point: 290°C).
Kinetic Analysis :
-
Cyclization occurs via intramolecular nucleophilic attack, favored by electron-donating methyl groups .
Polymerization
The maleimide double bond enables radical or anionic addition polymerization.
Radical Polymerization
-
Initiator : AIBN (azobisisobutyronitrile).
-
Product : Poly(this compound) with Mn ≈ 15,000 Da.
Thermal Stability :
Degradation in Geological Contexts
This compound is identified in Cretaceous/Tertiary boundary sediments as a diagenetic product of chlorophyll derivatives .
Sample Location | Concentration (nmol/g) | Dominant Isomer | Source |
---|---|---|---|
Kawaruppu claystone | 4–49 (bound form) | 2-Methyl-3-ethylmaleimide |
Environmental Relevance :
Aplicaciones Científicas De Investigación
Antifungal Applications
Antifungal Activity Against Pathogens
DMMI exhibits significant antifungal properties against various pathogens, notably Sclerotinia sclerotiorum, which is a common fungal pathogen affecting crops. Research indicates that DMMI inhibits the growth of this fungus effectively, making it a potential candidate for agricultural applications.
Compound | Target Pathogen | Activity |
---|---|---|
2,3-Dimethylmaleimide | Sclerotinia sclerotiorum | Significant inhibition |
Maleic Anhydride Derivatives | Botrytis cinerea | Moderate inhibition |
A study highlighted that various maleic anhydride derivatives, including DMMI, presented antifungal activity against Botrytis cinerea, indicating a broader spectrum of efficacy among related compounds .
Bioconjugation Techniques
Reactivity with Thiol Groups
DMMI is extensively used in bioconjugation due to its ability to react selectively with thiol groups in proteins. This property is crucial for the development of targeted drug delivery systems and protein labeling techniques.
- Case Study: Melittin Modification
A notable application involved modifying melittin (a peptide from bee venom) by introducing a pH-sensitive amide bond with DMMI. The modified peptide showed improved biocompatibility and targeted antitumor activity, demonstrating the potential for DMMI in therapeutic applications .
Bioconjugate | Modification Technique | Outcome |
---|---|---|
Melittin-DMMA | DMMI conjugation | Enhanced targeting and safety |
Polymer Chemistry
Role in Hydrogel Formation
DMMI is utilized as a monomer in the synthesis of hydrogels through UV-induced dimerization. This process allows for the creation of interconnected hydrogel networks with tailored mechanical properties suitable for biomedical applications.
- Inter-Crosslinking Mechanism
Research demonstrated that DMMI can interconnect acrylamide-based hydrogel blocks through dimerization upon UV irradiation. This method enhances the stability and functionality of hydrogels for applications such as drug delivery systems and tissue engineering .
Hydrogel Composition | DMMI Content (%) | Mechanical Stability |
---|---|---|
PAAm-G1a | 7.5 | High stability under motion |
PAAm-G1b | 9.0 | Increased dimerization rate |
Mecanismo De Acción
The mechanism of action of 2,3-dimethylmaleimide in antifungal applications involves the inhibition of fungal growth by interacting with specific enzymes and disrupting cellular processes. The hydrophobicity of the N-substituents in dimethylmaleimides is associated with their antifungal activity . In bioconjugation, the maleimide group reacts with thiol groups in proteins, forming stable thioether bonds .
Comparación Con Compuestos Similares
Reactivity in Photocycloaddition Reactions
DMMI undergoes [2+2] photocycloaddition with aromatic heterocycles like selenophene, forming 1:1 and 2:1 adducts. This contrasts with unsubstituted maleimide, which primarily forms 1:1 adducts due to steric limitations. The methyl groups in DMMI reduce steric hindrance during dimerization, enabling stable inter-crosslinking in hydrogels . In contrast, N-phenylmaleimide exhibits slower reaction kinetics due to bulkier substituents .
Table 1: Photocycloaddition Reactivity Comparison
Performance as Plasticizers
N-Substituted DMMI esters (e.g., benzoates, methacrylates) exhibit superior plasticizing efficiency for PVC compared to dioctyl phthalate (DOP) and dibutyl phthalate (DBP). As shown in Table V of , DMMI-based esters lower PVC softening points by 15–20°C at 0.4 g/g loading, whereas DOP reduces it by only 12°C. The rigidity of the maleimide ring enhances compatibility with PVC .
Table 2: Plasticizer Efficiency (Softening Point Reduction)
Compound | Softening Point Reduction (°C) |
---|---|
DMMI-based cinnamate [10] | 20 |
DOP | 12 |
DBP | 10 |
Thiol-Sensing Probes
DMMI derivatives (e.g., probes 54 and 55) exhibit fluorescence enhancement upon thiol addition. Probe 54 shows a quantum yield increase from 0.04 to 0.22 with mercaptopropionic acid (MPA), outperforming unsubstituted maleimide probes, which often suffer from solubility issues . The methyl groups in DMMI improve solubility in aqueous-DMSO mixtures, enabling practical applications in protein labeling .
Actividad Biológica
2,3-Dimethylmaleimide is an organic compound belonging to the maleimide family, characterized by its unique structure and diverse biological activities. This compound has garnered attention for its antifungal, antibacterial, and potential therapeutic properties. This article provides a comprehensive overview of the biological activity of this compound, highlighting its mechanisms of action, biochemical properties, and applications in scientific research.
Chemical Structure and Properties
This compound (CAS Number: 17825-86-4) is derived from maleic anhydride and exhibits a double bond characteristic of maleimides. The structure can be represented as follows:
This compound's reactivity is primarily due to the presence of the maleimide group, which interacts with thiol groups in proteins, making it valuable in bioconjugation applications.
Antifungal Activity
One of the most notable biological activities of this compound is its antifungal effect against Sclerotinia sclerotiorum, a significant pathogen affecting various crops. Research indicates that this compound inhibits fungal growth by interfering with cellular processes essential for fungal survival.
The antifungal activity is believed to stem from the compound's ability to disrupt cellular integrity and metabolism in fungi. The specific mode of action involves:
- Inhibition of Cell Wall Synthesis : this compound disrupts the synthesis of chitin and glucans in the fungal cell wall.
- Enzyme Inhibition : It has been shown to inhibit key enzymes involved in metabolic pathways critical for fungal growth.
Antibacterial Activity
In addition to its antifungal properties, this compound has demonstrated antibacterial activity , particularly against gram-positive bacteria. Studies have reported that maleimide derivatives exhibit varying degrees of inhibition against bacterial strains.
The biochemical properties of this compound include:
- Solubility : The compound is soluble in organic solvents such as DMSO and ethanol.
- Stability : It remains stable under physiological conditions but may undergo hydrolysis in aqueous environments.
Study on Fungal Biotransformation
A study conducted by Sortino et al. explored the biotransformation of various maleimides by filamentous fungi. The results indicated that this compound could be effectively reduced by Aspergillus species to yield succinimides with high conversion rates (up to 99%) . This highlights not only its antifungal potential but also its utility as a substrate for biocatalysis.
Fungal Strain | Conversion Rate (%) | Product Obtained |
---|---|---|
Aspergillus flavus | 96 | N-phenyl-2,3-dimethylsuccinimide |
Aspergillus niger | 98 | N-phenyl-2,3-dimethylsuccinimide |
Fusarium graminearum | 39 | N-phenyl-2,3-dimethylsuccinimide |
Anti-Leishmanial Activity
Recent studies have also investigated the anti-leishmanial properties of maleimides, including derivatives like this compound. These compounds exhibited significant activity against Leishmania donovani, with some showing IC50 values lower than standard treatments . This suggests a potential pathway for developing new anti-leishmanial drugs based on maleimide structures.
Applications in Research
The unique properties of this compound make it suitable for various applications:
- Bioconjugation : Its reactivity with thiols allows for targeted drug delivery systems.
- Polymer Chemistry : Used as a monomer in synthesizing polymers with specific functionalities.
- Antifungal Agents : Promising candidate for agricultural fungicides targeting crop pathogens.
Q & A
Basic Research Questions
Q. What are the established methods for synthesizing 2,3-Dimethylmaleimide and its derivatives?
Methodological Answer: this compound derivatives are typically synthesized via the reaction of substituted maleic anhydrides with primary amines. For example:
- Step 1: React 2,3-dimethylmaleic anhydride with an amine (e.g., aniline) in an aprotic solvent (e.g., toluene) under reflux conditions.
- Step 2: Purify the product via recrystallization or column chromatography.
- Key Considerations: Monitor reaction progress using thin-layer chromatography (TLC). Adjust stoichiometry and solvent polarity to optimize yield. Derivatives like 2-(dimethylmaleinimido)acrylamide (DMIAAm) can be synthesized by functionalizing acrylamide precursors .
Q. How is this compound characterized using spectroscopic techniques?
Methodological Answer:
- Nuclear Magnetic Resonance (NMR): Use and NMR to confirm substituent positions and purity. For example, maleimide carbonyl peaks typically appear at 170–175 ppm in NMR.
- Infrared Spectroscopy (IR): Identify maleimide C=O stretching vibrations (~1700 cm) and C-N-C bending (~690 cm).
- Mass Spectrometry (MS): Confirm molecular weight via high-resolution MS (HRMS).
- Reference Standards: Compare data with published spectra for validation .
Advanced Research Questions
Q. How can this compound be utilized in designing photocrosslinkable hydrogels for biomedical applications?
Methodological Answer: this compound’s [2+2] photocycloaddition reactivity enables hydrogel formation:
-
Functionalization: Incorporate dimethylmaleimide groups into polymers (e.g., polyacrylamide) via Michael addition or copolymerization.
-
Crosslinking: Expose the functionalized polymer to UV light (254–365 nm) to induce dimerization.
-
Optimization Parameters:
Parameter Impact on Hydrogel Properties Reference UV Wavelength Crosslinking efficiency and speed Polymer Concentration Mesh size and mechanical strength Irradiation Time Degree of crosslinking -
Applications: Tailor hydrogels for drug delivery or tissue engineering by adjusting crosslinking density .
Q. What experimental approaches are used to evaluate the antifungal activity of this compound derivatives?
Methodological Answer:
- Assay Design:
- Microdilution Assay: Prepare serial dilutions of the compound in fungal growth medium (e.g., potato dextrose broth). Inoculate with Botrytis cinerea spores.
- Controls: Include positive (e.g., fluconazole) and negative (solvent-only) controls.
- Endpoint Analysis: Measure minimum inhibitory concentration (MIC) after 48–72 hours.
- Statistical Validation: Use ANOVA to compare growth inhibition across concentrations.
- Reference Study: Li et al. (2012) demonstrated dose-dependent antifungal activity using similar protocols .
Q. How can researchers address contradictions in reported reactivity of this compound in Diels-Alder reactions?
Methodological Answer: Discrepancies often arise from reaction conditions or diene electronic properties.
- Controlled Experiments:
- Vary solvents (polar vs. nonpolar), temperatures, and diene structures (electron-rich vs. electron-poor).
- Use kinetic studies (e.g., NMR time-course) to track reaction progress.
- Computational Modeling: Apply density functional theory (DFT) to calculate activation energies and transition states.
- Data Reconciliation: Compare results under standardized conditions to isolate variables .
Q. What strategies optimize the stability of this compound during storage and experimental use?
Methodological Answer:
- Storage Conditions:
- Store in airtight, light-resistant containers under inert gas (e.g., argon) at –20°C.
- Add stabilizers (e.g., hydroquinone) to prevent radical-mediated degradation.
- Stability Monitoring:
- Use HPLC or TLC to detect decomposition products (e.g., maleic acid derivatives).
- Conduct accelerated aging studies at elevated temperatures to predict shelf life.
- Safety Precautions: Refer to handling guidelines for related anhydrides (e.g., 2,3-dimethylmaleic anhydride) .
Q. Data Presentation Guidelines
Table 1: Key Parameters for Antifungal Assays
Assay Type | Key Steps | Controls | Data Analysis | Reference |
---|---|---|---|---|
Microdilution | Serial dilution in broth | Positive (antifungal agent) | MIC determination | |
Disk Diffusion | Agar plate inoculation | Negative (solvent control) | Zone of inhibition |
Note: Ensure all data complies with journal standards for chemical manuscripts (e.g., limited structures in graphics, SI units) .
Propiedades
IUPAC Name |
3,4-dimethylpyrrole-2,5-dione | |
---|---|---|
Source | PubChem | |
URL | https://pubchem.ncbi.nlm.nih.gov | |
Description | Data deposited in or computed by PubChem | |
InChI |
InChI=1S/C6H7NO2/c1-3-4(2)6(9)7-5(3)8/h1-2H3,(H,7,8,9) | |
Source | PubChem | |
URL | https://pubchem.ncbi.nlm.nih.gov | |
Description | Data deposited in or computed by PubChem | |
InChI Key |
ZTWMBHJPUJJJME-UHFFFAOYSA-N | |
Source | PubChem | |
URL | https://pubchem.ncbi.nlm.nih.gov | |
Description | Data deposited in or computed by PubChem | |
Canonical SMILES |
CC1=C(C(=O)NC1=O)C | |
Source | PubChem | |
URL | https://pubchem.ncbi.nlm.nih.gov | |
Description | Data deposited in or computed by PubChem | |
Molecular Formula |
C6H7NO2 | |
Source | PubChem | |
URL | https://pubchem.ncbi.nlm.nih.gov | |
Description | Data deposited in or computed by PubChem | |
DSSTOX Substance ID |
DTXSID70170446 | |
Record name | 2,3-Dimethylmaleimide | |
Source | EPA DSSTox | |
URL | https://comptox.epa.gov/dashboard/DTXSID70170446 | |
Description | DSSTox provides a high quality public chemistry resource for supporting improved predictive toxicology. | |
Molecular Weight |
125.13 g/mol | |
Source | PubChem | |
URL | https://pubchem.ncbi.nlm.nih.gov | |
Description | Data deposited in or computed by PubChem | |
CAS No. |
17825-86-4 | |
Record name | 2,3-Dimethylmaleimide | |
Source | ChemIDplus | |
URL | https://pubchem.ncbi.nlm.nih.gov/substance/?source=chemidplus&sourceid=0017825864 | |
Description | ChemIDplus is a free, web search system that provides access to the structure and nomenclature authority files used for the identification of chemical substances cited in National Library of Medicine (NLM) databases, including the TOXNET system. | |
Record name | 2,3-Dimethylmaleimide | |
Source | EPA DSSTox | |
URL | https://comptox.epa.gov/dashboard/DTXSID70170446 | |
Description | DSSTox provides a high quality public chemistry resource for supporting improved predictive toxicology. | |
Record name | 2,3-DIMETHYLMALEIMIDE | |
Source | FDA Global Substance Registration System (GSRS) | |
URL | https://gsrs.ncats.nih.gov/ginas/app/beta/substances/56B9BV5BAR | |
Description | The FDA Global Substance Registration System (GSRS) enables the efficient and accurate exchange of information on what substances are in regulated products. Instead of relying on names, which vary across regulatory domains, countries, and regions, the GSRS knowledge base makes it possible for substances to be defined by standardized, scientific descriptions. | |
Explanation | Unless otherwise noted, the contents of the FDA website (www.fda.gov), both text and graphics, are not copyrighted. They are in the public domain and may be republished, reprinted and otherwise used freely by anyone without the need to obtain permission from FDA. Credit to the U.S. Food and Drug Administration as the source is appreciated but not required. | |
Retrosynthesis Analysis
AI-Powered Synthesis Planning: Our tool employs the Template_relevance Pistachio, Template_relevance Bkms_metabolic, Template_relevance Pistachio_ringbreaker, Template_relevance Reaxys, Template_relevance Reaxys_biocatalysis model, leveraging a vast database of chemical reactions to predict feasible synthetic routes.
One-Step Synthesis Focus: Specifically designed for one-step synthesis, it provides concise and direct routes for your target compounds, streamlining the synthesis process.
Accurate Predictions: Utilizing the extensive PISTACHIO, BKMS_METABOLIC, PISTACHIO_RINGBREAKER, REAXYS, REAXYS_BIOCATALYSIS database, our tool offers high-accuracy predictions, reflecting the latest in chemical research and data.
Strategy Settings
Precursor scoring | Relevance Heuristic |
---|---|
Min. plausibility | 0.01 |
Model | Template_relevance |
Template Set | Pistachio/Bkms_metabolic/Pistachio_ringbreaker/Reaxys/Reaxys_biocatalysis |
Top-N result to add to graph | 6 |
Feasible Synthetic Routes
Descargo de responsabilidad e información sobre productos de investigación in vitro
Tenga en cuenta que todos los artículos e información de productos presentados en BenchChem están destinados únicamente con fines informativos. Los productos disponibles para la compra en BenchChem están diseñados específicamente para estudios in vitro, que se realizan fuera de organismos vivos. Los estudios in vitro, derivados del término latino "in vidrio", involucran experimentos realizados en entornos de laboratorio controlados utilizando células o tejidos. Es importante tener en cuenta que estos productos no se clasifican como medicamentos y no han recibido la aprobación de la FDA para la prevención, tratamiento o cura de ninguna condición médica, dolencia o enfermedad. Debemos enfatizar que cualquier forma de introducción corporal de estos productos en humanos o animales está estrictamente prohibida por ley. Es esencial adherirse a estas pautas para garantizar el cumplimiento de los estándares legales y éticos en la investigación y experimentación.