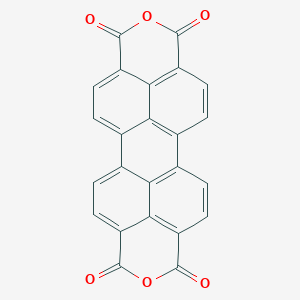
3,4,9,10-Tetracarboxilato de perileno dianhidrido
Descripción general
Descripción
3,4,9,10-Perylenetetracarboxylic dianhydride, also known as perylenetetracarboxylic acid dianhydride, is a large π-conjugated semiconductor aromatic organic molecule. It is an organic dye molecule and an organic semiconductor. It is a dark red solid with low solubility in aromatic solvents and has attracted significant interest as an organic semiconductor .
Aplicaciones Científicas De Investigación
3,4,9,10-Perylenetetracarboxylic dianhydride has a wide range of scientific research applications. In chemistry, it is used as a precursor for the synthesis of rylene dyes and pigments . In biology, it has been explored for its potential use in bioimaging and as a fluorescent probe . In medicine, the compound’s derivatives have been investigated for their potential use in drug delivery systems and as anticancer agents . In industry, it is used in the production of organic semiconductors, which are essential components in organic electronics and optoelectronic devices . It is also used in the fabrication of carbon dots for anticounterfeiting applications .
Mecanismo De Acción
Target of Action
The primary targets of PTCDA are glucose oxidase (GOx) and catalase (CAT). These enzymes play crucial roles in the metabolism of glucose and the breakdown of hydrogen peroxide in cells, respectively .
Mode of Action
PTCDA exhibits both glucose oxidase-like and catalase-like activities. This is likely due to the highly conjugated structure and high electron mobility of PTCDA . The compound interacts with its targets, mimicking their activities and inducing changes in the biochemical processes they are involved in.
Biochemical Pathways
The affected pathways primarily involve glucose metabolism and hydrogen peroxide breakdown. By mimicking the activities of GOx and CAT, PTCDA influences these pathways and their downstream effects, which include energy production and the neutralization of harmful reactive oxygen species .
Result of Action
The molecular and cellular effects of PTCDA’s action are related to its enzyme-like activities. By mimicking GOx and CAT, PTCDA can influence cellular energy production and the neutralization of harmful substances. This can have various effects on cellular function and health .
Análisis Bioquímico
Biochemical Properties
3,4,9,10-Perylenetetracarboxylic dianhydride exhibits both glucose oxidase (GOx)‐like and catalase (CAT)‐like activities . This may be due to the fact that 3,4,9,10-Perylenetetracarboxylic dianhydride possesses the features of a highly conjugated structure and high electron mobility .
Molecular Mechanism
The molecular mechanism of 3,4,9,10-Perylenetetracarboxylic dianhydride involves its interaction with glucose oxidase and catalase .
Métodos De Preparación
3,4,9,10-Perylenetetracarboxylic dianhydride can be synthesized through various methods. One common method involves the condensation of perylene-3,4,9,10-tetracarboxylic acid with acetic anhydride . The reaction typically occurs under reflux conditions, and the product is purified through recrystallization. Industrial production methods often involve similar synthetic routes but are optimized for large-scale production . Another method involves the solvothermal approach, where the compound is derived from perylene-3,4,9,10-tetracarboxylic dianhydride and subsequently purified using column chromatography .
Análisis De Reacciones Químicas
3,4,9,10-Perylenetetracarboxylic dianhydride undergoes various chemical reactions, including hydrolysis, complexation, and redox reactions. For instance, it can be hydrolyzed to form perylenetetracarboxylic acid . It also forms complexes with metal ions such as nickel, cobalt, and manganese, which are used in the development of novel organic electrode materials for lithium-ion batteries . Additionally, the compound participates in redox reactions, such as the multivalent aluminium-ion redox mechanism, which involves the intercalation of aluminium ions .
Comparación Con Compuestos Similares
3,4,9,10-Perylenetetracarboxylic dianhydride can be compared with other similar compounds, such as 1,4,5,8-naphthalenetetracarboxylic dianhydride and pyromellitic dianhydride . These compounds share similar structural features and are used in similar applications, such as the production of organic semiconductors and dyes . 3,4,9,10-perylenetetracarboxylic dianhydride is unique due to its larger π-conjugated system, which results in higher electron mobility and better performance in electronic applications .
Similar Compounds::Propiedades
IUPAC Name |
7,18-dioxaheptacyclo[14.6.2.22,5.03,12.04,9.013,23.020,24]hexacosa-1(23),2,4,9,11,13,15,20(24),21,25-decaene-6,8,17,19-tetrone | |
---|---|---|
Source | PubChem | |
URL | https://pubchem.ncbi.nlm.nih.gov | |
Description | Data deposited in or computed by PubChem | |
InChI |
InChI=1S/C24H8O6/c25-21-13-5-1-9-10-2-6-15-20-16(24(28)30-23(15)27)8-4-12(18(10)20)11-3-7-14(22(26)29-21)19(13)17(9)11/h1-8H | |
Source | PubChem | |
URL | https://pubchem.ncbi.nlm.nih.gov | |
Description | Data deposited in or computed by PubChem | |
InChI Key |
CLYVDMAATCIVBF-UHFFFAOYSA-N | |
Source | PubChem | |
URL | https://pubchem.ncbi.nlm.nih.gov | |
Description | Data deposited in or computed by PubChem | |
Canonical SMILES |
C1=CC2=C3C(=CC=C4C3=C1C5=C6C4=CC=C7C6=C(C=C5)C(=O)OC7=O)C(=O)OC2=O | |
Source | PubChem | |
URL | https://pubchem.ncbi.nlm.nih.gov | |
Description | Data deposited in or computed by PubChem | |
Molecular Formula |
C24H8O6 | |
Source | PubChem | |
URL | https://pubchem.ncbi.nlm.nih.gov | |
Description | Data deposited in or computed by PubChem | |
Related CAS |
95658-90-5 | |
Record name | Perylo[3,4-cd:9,10-c'd′]dipyran-1,3,8,10-tetrone, homopolymer | |
Source | CAS Common Chemistry | |
URL | https://commonchemistry.cas.org/detail?cas_rn=95658-90-5 | |
Description | CAS Common Chemistry is an open community resource for accessing chemical information. Nearly 500,000 chemical substances from CAS REGISTRY cover areas of community interest, including common and frequently regulated chemicals, and those relevant to high school and undergraduate chemistry classes. This chemical information, curated by our expert scientists, is provided in alignment with our mission as a division of the American Chemical Society. | |
Explanation | The data from CAS Common Chemistry is provided under a CC-BY-NC 4.0 license, unless otherwise stated. | |
DSSTOX Substance ID |
DTXSID1059577 | |
Record name | Perylo[3,4-cd:9,10-c'd']dipyran-1,3,8,10-tetrone | |
Source | EPA DSSTox | |
URL | https://comptox.epa.gov/dashboard/DTXSID1059577 | |
Description | DSSTox provides a high quality public chemistry resource for supporting improved predictive toxicology. | |
Molecular Weight |
392.3 g/mol | |
Source | PubChem | |
URL | https://pubchem.ncbi.nlm.nih.gov | |
Description | Data deposited in or computed by PubChem | |
Physical Description |
Dark red powder; [Sigma-Aldrich MSDS] | |
Record name | Perylene-3,4,9,10-tetracarboxylic dianhydride | |
Source | Haz-Map, Information on Hazardous Chemicals and Occupational Diseases | |
URL | https://haz-map.com/Agents/20388 | |
Description | Haz-Map® is an occupational health database designed for health and safety professionals and for consumers seeking information about the adverse effects of workplace exposures to chemical and biological agents. | |
Explanation | Copyright (c) 2022 Haz-Map(R). All rights reserved. Unless otherwise indicated, all materials from Haz-Map are copyrighted by Haz-Map(R). No part of these materials, either text or image may be used for any purpose other than for personal use. Therefore, reproduction, modification, storage in a retrieval system or retransmission, in any form or by any means, electronic, mechanical or otherwise, for reasons other than personal use, is strictly prohibited without prior written permission. | |
CAS No. |
128-69-8 | |
Record name | 3,4,9,10-Perylenetetracarboxylic dianhydride | |
Source | CAS Common Chemistry | |
URL | https://commonchemistry.cas.org/detail?cas_rn=128-69-8 | |
Description | CAS Common Chemistry is an open community resource for accessing chemical information. Nearly 500,000 chemical substances from CAS REGISTRY cover areas of community interest, including common and frequently regulated chemicals, and those relevant to high school and undergraduate chemistry classes. This chemical information, curated by our expert scientists, is provided in alignment with our mission as a division of the American Chemical Society. | |
Explanation | The data from CAS Common Chemistry is provided under a CC-BY-NC 4.0 license, unless otherwise stated. | |
Record name | Pigment Red 224 | |
Source | ChemIDplus | |
URL | https://pubchem.ncbi.nlm.nih.gov/substance/?source=chemidplus&sourceid=0000128698 | |
Description | ChemIDplus is a free, web search system that provides access to the structure and nomenclature authority files used for the identification of chemical substances cited in National Library of Medicine (NLM) databases, including the TOXNET system. | |
Record name | 128-69-8 | |
Source | DTP/NCI | |
URL | https://dtp.cancer.gov/dtpstandard/servlet/dwindex?searchtype=NSC&outputformat=html&searchlist=79895 | |
Description | The NCI Development Therapeutics Program (DTP) provides services and resources to the academic and private-sector research communities worldwide to facilitate the discovery and development of new cancer therapeutic agents. | |
Explanation | Unless otherwise indicated, all text within NCI products is free of copyright and may be reused without our permission. Credit the National Cancer Institute as the source. | |
Record name | Perylo[3,4-cd:9,10-c'd']dipyran-1,3,8,10-tetrone | |
Source | EPA Chemicals under the TSCA | |
URL | https://www.epa.gov/chemicals-under-tsca | |
Description | EPA Chemicals under the Toxic Substances Control Act (TSCA) collection contains information on chemicals and their regulations under TSCA, including non-confidential content from the TSCA Chemical Substance Inventory and Chemical Data Reporting. | |
Record name | Perylo[3,4-cd:9,10-c'd']dipyran-1,3,8,10-tetrone | |
Source | EPA DSSTox | |
URL | https://comptox.epa.gov/dashboard/DTXSID1059577 | |
Description | DSSTox provides a high quality public chemistry resource for supporting improved predictive toxicology. | |
Record name | Perylene-3,4:9,10-tetracarboxylic dianhydride | |
Source | European Chemicals Agency (ECHA) | |
URL | https://echa.europa.eu/substance-information/-/substanceinfo/100.004.461 | |
Description | The European Chemicals Agency (ECHA) is an agency of the European Union which is the driving force among regulatory authorities in implementing the EU's groundbreaking chemicals legislation for the benefit of human health and the environment as well as for innovation and competitiveness. | |
Explanation | Use of the information, documents and data from the ECHA website is subject to the terms and conditions of this Legal Notice, and subject to other binding limitations provided for under applicable law, the information, documents and data made available on the ECHA website may be reproduced, distributed and/or used, totally or in part, for non-commercial purposes provided that ECHA is acknowledged as the source: "Source: European Chemicals Agency, http://echa.europa.eu/". Such acknowledgement must be included in each copy of the material. ECHA permits and encourages organisations and individuals to create links to the ECHA website under the following cumulative conditions: Links can only be made to webpages that provide a link to the Legal Notice page. | |
Record name | PIGMENT RED 224 | |
Source | FDA Global Substance Registration System (GSRS) | |
URL | https://gsrs.ncats.nih.gov/ginas/app/beta/substances/NH27FW2PET | |
Description | The FDA Global Substance Registration System (GSRS) enables the efficient and accurate exchange of information on what substances are in regulated products. Instead of relying on names, which vary across regulatory domains, countries, and regions, the GSRS knowledge base makes it possible for substances to be defined by standardized, scientific descriptions. | |
Explanation | Unless otherwise noted, the contents of the FDA website (www.fda.gov), both text and graphics, are not copyrighted. They are in the public domain and may be republished, reprinted and otherwise used freely by anyone without the need to obtain permission from FDA. Credit to the U.S. Food and Drug Administration as the source is appreciated but not required. | |
Synthesis routes and methods
Procedure details
Retrosynthesis Analysis
AI-Powered Synthesis Planning: Our tool employs the Template_relevance Pistachio, Template_relevance Bkms_metabolic, Template_relevance Pistachio_ringbreaker, Template_relevance Reaxys, Template_relevance Reaxys_biocatalysis model, leveraging a vast database of chemical reactions to predict feasible synthetic routes.
One-Step Synthesis Focus: Specifically designed for one-step synthesis, it provides concise and direct routes for your target compounds, streamlining the synthesis process.
Accurate Predictions: Utilizing the extensive PISTACHIO, BKMS_METABOLIC, PISTACHIO_RINGBREAKER, REAXYS, REAXYS_BIOCATALYSIS database, our tool offers high-accuracy predictions, reflecting the latest in chemical research and data.
Strategy Settings
Precursor scoring | Relevance Heuristic |
---|---|
Min. plausibility | 0.01 |
Model | Template_relevance |
Template Set | Pistachio/Bkms_metabolic/Pistachio_ringbreaker/Reaxys/Reaxys_biocatalysis |
Top-N result to add to graph | 6 |
Feasible Synthetic Routes
Q1: What is the molecular formula and weight of 3,4,9,10-perylenetetracarboxylic dianhydride?
A1: The molecular formula of PTCDA is C24H8O6, and its molecular weight is 392.32 g/mol.
Q2: Which spectroscopic techniques are commonly used to characterize PTCDA?
A2: Researchers frequently utilize various spectroscopic methods to characterize PTCDA, including:
- Fourier-transform infrared spectroscopy (FT-IR) [, , ] to analyze the vibrational modes and identify functional groups.
- Raman spectroscopy [, , , , ] to investigate vibrational and rotational modes, providing complementary information to FT-IR.
- X-ray photoelectron spectroscopy (XPS) [, , , ] to determine elemental composition and chemical states within the material.
- Ultraviolet-visible spectroscopy (UV-Vis) [, , , , ] to study the electronic transitions and determine optical band gap.
- Near-edge X-ray absorption fine structure (NEXAFS) [, , ] to investigate the unoccupied electronic states and molecular orientation.
Q3: What is the significance of the herringbone structure observed in PTCDA crystals?
A3: The herringbone packing motif of PTCDA molecules within its crystal structure significantly impacts its properties. This arrangement leads to strong intermolecular interactions, resulting in a high melting point and low solubility in common solvents []. This structure also influences charge transport and optical properties. [] demonstrated that Mg-ion insertion in a PTCDA electrode caused unique squeezing deformation of this herringbone structure.
Q4: How does PTCDA interact with different substrates?
A4: PTCDA exhibits diverse interactions with different substrates, influencing its growth mode and electronic properties:
- Weak Interaction: On inert substrates like highly oriented pyrolytic graphite (HOPG) [] and Ag(111) [], PTCDA molecules experience weak van der Waals forces, resulting in well-ordered layers with a flat-lying orientation.
- Stronger Interaction: On reactive surfaces like Si(100) [, ] and GaAs(100) [, ], PTCDA shows a stronger interaction, potentially involving chemical bonding, leading to different growth modes and potential interface dipoles.
- Metal Contacts: The interaction of PTCDA with various metals, including Au [, ], Ag [, , ], and Al [, ], is crucial for device performance. The choice of metal influences charge injection barriers and interface dipole formation.
Q5: What are the advantages of using PTCDA in organic electronics?
A5: PTCDA is a promising organic semiconductor due to its several attractive features:
- High Electron Affinity: This allows efficient electron injection and transport, making it suitable for n-type semiconductor applications. [, ]
- Thermal Stability: PTCDA exhibits high thermal stability, making it compatible with various device fabrication processes. [, ]
- Film Formation: Its ability to form well-ordered thin films through vacuum deposition techniques is crucial for device fabrication. [, , ]
Q6: How does the solubility of PTCDA affect its applications?
A6: The limited solubility of PTCDA in common solvents poses challenges for solution-based processing techniques. Researchers have explored different strategies to overcome this limitation:
- Chemical Modification: Introducing functional groups to the PTCDA molecule can improve its solubility. []
- Composite Formation: Incorporating PTCDA into composite materials with other materials, like graphene [], can enhance its processability while preserving its desirable properties.
Q7: How is PTCDA used in organic light-emitting diodes (OLEDs)?
A7: PTCDA has been explored as an electron transport layer in OLED devices due to its high electron affinity. [] demonstrated enhanced performance in OLEDs incorporating lithium carbonate doped PTCDA, highlighting its potential for improving electron injection.
Q8: How does PTCDA contribute to the performance of organic solar cells?
A8: PTCDA is often used as an electron acceptor material in organic solar cells. Its energy level alignment with donor materials like CuPc allows efficient exciton dissociation at the interface, leading to charge separation and current generation. [, , ]
Q9: Can PTCDA be used in lithium-ion batteries?
A9: Yes, PTCDA has been investigated as a potential electrode material for lithium-ion batteries. [] showed promising results using a PTCDA/carbon nanotube nanocomposite as a cathode material, demonstrating improved rate capability and cycling stability. Additionally, [] investigated PTCDA as an electrode material for both lithium-ion batteries (LIBs) and sodium-ion batteries (SIBs) when incorporated in MXene@PTCDA heterostructures.
Q10: Are there any environmental concerns regarding PTCDA?
A10: While PTCDA itself may not pose significant environmental risks, understanding its degradation pathways and potential ecotoxicological effects is crucial. Further research is necessary to develop effective recycling and waste management strategies for PTCDA-containing devices. []
Q11: How is computational chemistry used to study PTCDA?
A11: Computational chemistry plays a vital role in understanding and predicting the properties of PTCDA. Density functional theory (DFT) calculations are commonly employed to investigate its electronic structure, interactions with different substrates, and charge transport mechanisms. [, , , , , ]
Q12: What insights can be gained from molecular dynamics (MD) simulations of PTCDA?
A12: MD simulations are valuable for studying the dynamic behavior of PTCDA molecules. These simulations can provide insights into its self-assembly processes on surfaces, interactions with other molecules in solutions, and the diffusion of dopants within its crystal structure. []
Q13: What are some exciting research directions for PTCDA?
A13: Ongoing research on PTCDA focuses on:
- Improving Device Performance: Optimizing PTCDA-based devices like OLEDs and solar cells by tuning its morphology, interfaces, and charge transport properties. [, , ]
- Exploring New Applications: Investigating PTCDA's potential in emerging fields like thermoelectrics [] and bioelectronics.
- Developing Sustainable Solutions: Addressing the environmental impact of PTCDA by exploring its biodegradability, developing efficient recycling methods, and investigating sustainable alternatives. []
Descargo de responsabilidad e información sobre productos de investigación in vitro
Tenga en cuenta que todos los artículos e información de productos presentados en BenchChem están destinados únicamente con fines informativos. Los productos disponibles para la compra en BenchChem están diseñados específicamente para estudios in vitro, que se realizan fuera de organismos vivos. Los estudios in vitro, derivados del término latino "in vidrio", involucran experimentos realizados en entornos de laboratorio controlados utilizando células o tejidos. Es importante tener en cuenta que estos productos no se clasifican como medicamentos y no han recibido la aprobación de la FDA para la prevención, tratamiento o cura de ninguna condición médica, dolencia o enfermedad. Debemos enfatizar que cualquier forma de introducción corporal de estos productos en humanos o animales está estrictamente prohibida por ley. Es esencial adherirse a estas pautas para garantizar el cumplimiento de los estándares legales y éticos en la investigación y experimentación.