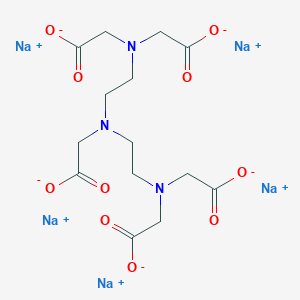
Pentasodio DTPA
Descripción general
Descripción
Pentasodium diethylenetriaminepentaacetate is a useful research compound. Its molecular formula is C14H23N3NaO10 and its molecular weight is 416.34 g/mol. The purity is usually 95%.
The exact mass of the compound Pentasodium diethylenetriaminepentaacetate is unknown and the complexity rating of the compound is unknown. The United Nations designated GHS hazard class pictogram is Irritant;Health Hazard, and the GHS signal word is WarningThe storage condition is unknown. Please store according to label instructions upon receipt of goods.Use and application categories indicated by third-party sources: Cosmetics -> Chelating. However, this does not mean our product can be used or applied in the same or a similar way.
BenchChem offers high-quality Pentasodium diethylenetriaminepentaacetate suitable for many research applications. Different packaging options are available to accommodate customers' requirements. Please inquire for more information about Pentasodium diethylenetriaminepentaacetate including the price, delivery time, and more detailed information at info@benchchem.com.
Aplicaciones Científicas De Investigación
Tratamiento de la Anosmia Post COVID-19
El Pentasodio DTPA se ha utilizado en un ensayo clínico para tratar la anosmia (pérdida del olfato) en pacientes con COVID-19 post . El estudio encontró que la reducción de los cationes de calcio intranasales libres utilizando quelantes tópicos como el this compound podría conducir a la restauración de la función olfativa en pacientes con anosmia post-COVID-19 .
Agente Quelante para Iones Metálicos
El this compound se utiliza principalmente como agente quelante para la complejación y secuestro de iones metálicos . Como agente quelante, el DTPA se envuelve alrededor de un ion metálico formando hasta ocho enlaces .
Tratamiento de Materiales Radiactivos
El this compound se ha considerado para el tratamiento de materiales radiactivos como el plutonio, el americio y otros actínidos . En teoría, estos complejos son más propensos a ser eliminados en la orina .
Uso en Presencia de Oxidantes
El this compound debe considerarse para su uso cuando el quelante se utilizará en presencia de oxidantes como el peróxido .
Formación de Quelatos Metálicos Estables o Solubles
Cuando se buscan quelatos metálicos de mayor estabilidad o solubilidad, se debe considerar el this compound .
Mecanismo De Acción
Pentasodium DTPA, also known as Pentasodium diethylenetriaminepentaacetate or PENTASODIUM PENTETATE, is a synthetic polyamino carboxylic acid . Here is an overview of its mechanism of action:
Target of Action
Pentasodium DTPA’s primary targets are metal ions , specifically transuranic radionuclides such as plutonium, americium, and curium . It has eight coordinate bond-forming sites that can sequester these metal ions and form highly stable DTPA-metal ion complexes .
Mode of Action
The calcium and zinc trisodium salts of DTPA achieve their therapeutic potential by exchanging calcium and zinc cations with transuranic radionuclides . This exchange forms higher affinity complexes, which then promote the elimination of these radionuclides by glomerular filtration into the urine .
Biochemical Pathways
The biochemical pathways affected by Pentasodium DTPA primarily involve the chelation and elimination of metal ions . By forming stable complexes with these ions, DTPA prevents them from participating in harmful biochemical reactions within the body .
Pharmacokinetics
Pentasodium DTPA is eliminated from the body through the kidneys . Its pharmacokinetic properties allow it to increase the rates of elimination of internally deposited radionuclides . This is particularly useful in medical imaging and the treatment of internal contamination .
Result of Action
The result of Pentasodium DTPA’s action is the increased elimination of harmful radionuclides from the body . This reduces the committed effective dose of ionizing radiation delivered to tissues, thereby mitigating the acute and long-term health consequences of internal contamination .
Action Environment
The action of Pentasodium DTPA can be influenced by environmental factors. For instance, it is used in the presence of oxidizers such as peroxide when metal chelates of greater stability or solubility are sought . Additionally, it is typically administered as the calcium or zinc salt (Ca or Zn-DTPA), as these ions are readily displaced by more highly charged cations .
Análisis Bioquímico
Biochemical Properties
Pentasodium DTPA has a high affinity for metal cations . As a chelating agent, it wraps around a metal ion by forming up to eight bonds . Its complexes can also have an extra water molecule that coordinates the metal ion .
Cellular Effects
Pentasodium DTPA is used in medical imaging and for the decorporation of internally deposited radionuclides . It is FDA approved for the treatment of individuals with known or suspected internal contamination with plutonium, americium, or curium to increase the rates of elimination .
Molecular Mechanism
The calcium and zinc trisodium salts of Pentasodium DTPA achieve their therapeutic potential by exchanging calcium and zinc cations with transuranic radionuclides to form higher affinity complexes and then promote their elimination by glomerular filtration into the urine .
Temporal Effects in Laboratory Settings
In a 4-week dermal toxicity study, daily topical application of 0.05% Pentasodium DTPA to shaved and abraded rabbit skin produced moderate erythema after the first week and throughout the study, but no systemic toxicity .
Dosage Effects in Animal Models
The no observed adverse effect level (NOAEL) for rats given 40% Pentasodium DTPA by oral gavage was reported to be 83 mg/kg day−1 . The no observed effect level (NOEL) for maternal toxicity in pregnant rats was 400 mg/kg body weight and for fetal toxicity was 100 mg/kg body weight .
Propiedades
Número CAS |
140-01-2 |
---|---|
Fórmula molecular |
C14H23N3NaO10 |
Peso molecular |
416.34 g/mol |
Nombre IUPAC |
pentasodium;2-[bis[2-[bis(carboxylatomethyl)amino]ethyl]amino]acetate |
InChI |
InChI=1S/C14H23N3O10.Na/c18-10(19)5-15(1-3-16(6-11(20)21)7-12(22)23)2-4-17(8-13(24)25)9-14(26)27;/h1-9H2,(H,18,19)(H,20,21)(H,22,23)(H,24,25)(H,26,27); |
Clave InChI |
HVLGAHRQYRRROV-UHFFFAOYSA-N |
SMILES |
C(CN(CC(=O)[O-])CC(=O)[O-])N(CCN(CC(=O)[O-])CC(=O)[O-])CC(=O)[O-].[Na+].[Na+].[Na+].[Na+].[Na+] |
SMILES canónico |
C(CN(CC(=O)O)CC(=O)O)N(CCN(CC(=O)O)CC(=O)O)CC(=O)O.[Na] |
Key on ui other cas no. |
140-01-2 |
Descripción física |
DryPowder; Liquid |
Pictogramas |
Irritant; Health Hazard |
Números CAS relacionados |
13078-36-9 7578-43-0 |
Origen del producto |
United States |
Synthesis routes and methods
Procedure details
Retrosynthesis Analysis
AI-Powered Synthesis Planning: Our tool employs the Template_relevance Pistachio, Template_relevance Bkms_metabolic, Template_relevance Pistachio_ringbreaker, Template_relevance Reaxys, Template_relevance Reaxys_biocatalysis model, leveraging a vast database of chemical reactions to predict feasible synthetic routes.
One-Step Synthesis Focus: Specifically designed for one-step synthesis, it provides concise and direct routes for your target compounds, streamlining the synthesis process.
Accurate Predictions: Utilizing the extensive PISTACHIO, BKMS_METABOLIC, PISTACHIO_RINGBREAKER, REAXYS, REAXYS_BIOCATALYSIS database, our tool offers high-accuracy predictions, reflecting the latest in chemical research and data.
Strategy Settings
Precursor scoring | Relevance Heuristic |
---|---|
Min. plausibility | 0.01 |
Model | Template_relevance |
Template Set | Pistachio/Bkms_metabolic/Pistachio_ringbreaker/Reaxys/Reaxys_biocatalysis |
Top-N result to add to graph | 6 |
Feasible Synthetic Routes
Q1: What is the primary function of Pentasodium Pentetate in cosmetic formulations?
A1: Pentasodium Pentetate acts as a chelating agent in cosmetics. [] It achieves this by removing calcium and magnesium ions, which can negatively affect product performance. For instance, these ions can hinder foaming and cleansing, and also cause cloudiness in otherwise clear cosmetic solutions. []
Q2: Are there safety concerns regarding the use of Pentasodium Pentetate in cosmetics?
A2: Several studies suggest that Pentasodium Pentetate is safe for cosmetic use at current concentrations. Acute oral and dermal toxicity studies in rats revealed high LD50 values, indicating low toxicity. [] Additionally, clinical tests showed it to be non-irritating to moderately irritating and a non-sensitizer. [] Importantly, its poor skin penetration further minimizes potential systemic exposure. []
Q3: Has the safety of Pentasodium Pentetate been compared to other chelating agents used in cosmetics?
A3: Yes, the safety profile of Pentasodium Pentetate appears comparable to other approved chelating agents. Similar to EDTA and its salts, Pentasodium Pentetate has been deemed safe for cosmetic use. [] Other chelating agents, including Meta-, Tri-, and Hexam-etaphosphate salts, and Metasilicate salts (when formulated to avoid irritation), have also been found safe for such applications. []
Q4: Is there a specific application where Pentasodium Pentetate proves particularly effective?
A4: Pentasodium Pentetate finds significant use in hair dyes and colors, with concentrations ranging from 0.1% to 1.0%. [] This suggests its effectiveness in chelating metal ions that may interfere with the dyeing process or color stability in these products.
Q5: Beyond cosmetics, are there other known applications for Pentasodium Pentetate?
A5: Yes, research highlights its utility in separating americium and curium from lanthanide elements. [] In the "Talspeak" process, Pentasodium diethylenetriaminepentaacetate (Na5DTPA) selectively complexes with trivalent actinides. This allows for the efficient extraction of lanthanides using an organophosphate, effectively separating these elements. []
Descargo de responsabilidad e información sobre productos de investigación in vitro
Tenga en cuenta que todos los artículos e información de productos presentados en BenchChem están destinados únicamente con fines informativos. Los productos disponibles para la compra en BenchChem están diseñados específicamente para estudios in vitro, que se realizan fuera de organismos vivos. Los estudios in vitro, derivados del término latino "in vidrio", involucran experimentos realizados en entornos de laboratorio controlados utilizando células o tejidos. Es importante tener en cuenta que estos productos no se clasifican como medicamentos y no han recibido la aprobación de la FDA para la prevención, tratamiento o cura de ninguna condición médica, dolencia o enfermedad. Debemos enfatizar que cualquier forma de introducción corporal de estos productos en humanos o animales está estrictamente prohibida por ley. Es esencial adherirse a estas pautas para garantizar el cumplimiento de los estándares legales y éticos en la investigación y experimentación.