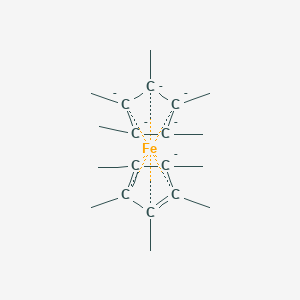
Bis(pentamethylcyclopentadienyl)iron(II)
- Haga clic en CONSULTA RÁPIDA para recibir una cotización de nuestro equipo de expertos.
- Con productos de calidad a un precio COMPETITIVO, puede centrarse más en su investigación.
Descripción general
Descripción
Bis(pentamethylcyclopentadienyl)iron(II), also known as decamethylferrocene, is a chemical compound with the formula Fe(C₅(CH₃)₅)₂. It is a member of the metallocene family, which consists of sandwich compounds where a metal atom is sandwiched between two cyclopentadienyl anions. This compound is notable for its stability and unique electronic properties, making it a valuable subject of study in various scientific fields .
Métodos De Preparación
Synthetic Routes and Reaction Conditions
Bis(pentamethylcyclopentadienyl)iron(II) is typically synthesized through the reaction of pentamethylcyclopentadiene with iron(II) chloride. The reaction proceeds as follows: [ 2 \text{Li(C}_5\text{Me}_5) + \text{FeCl}_2 \rightarrow \text{Fe(C}_5\text{Me}_5)_2 + 2 \text{LiCl} ] The product can be purified by sublimation .
Industrial Production Methods
While the industrial production methods for bis(pentamethylcyclopentadienyl)iron(II) are not extensively documented, the synthesis generally follows the same principles as laboratory methods, with adjustments for scale and efficiency. The use of inert atmospheres and controlled temperatures is crucial to ensure the purity and stability of the compound .
Análisis De Reacciones Químicas
Types of Reactions
Bis(pentamethylcyclopentadienyl)iron(II) undergoes various types of chemical reactions, including:
Oxidation: The compound can be oxidized to form decamethylferrocenium, a stable cation.
Reduction: It can act as a reducing agent in certain reactions.
Substitution: The pentamethylcyclopentadienyl ligands can be substituted under specific conditions.
Common Reagents and Conditions
Common reagents used in reactions with bis(pentamethylcyclopentadienyl)iron(II) include oxidizing agents like ferric chloride and reducing agents such as lithium aluminum hydride. Reactions are typically conducted under inert atmospheres to prevent unwanted side reactions .
Major Products
The major products formed from reactions involving bis(pentamethylcyclopentadienyl)iron(II) depend on the specific reaction conditions. For example, oxidation yields decamethylferrocenium, while substitution reactions can produce a variety of substituted metallocenes .
Aplicaciones Científicas De Investigación
Bis(pentamethylcyclopentadienyl)iron(II) has a wide range of applications in scientific research:
Chemistry: It is used as a reference standard in redox titrations and as a catalyst in various organic reactions.
Biology: The compound’s stability and electronic properties make it useful in studying electron transfer processes in biological systems.
Medicine: Research is ongoing into its potential use in drug delivery systems and as a component in diagnostic tools.
Industry: It is used in the production of high-purity graphene and other advanced materials
Mecanismo De Acción
The mechanism by which bis(pentamethylcyclopentadienyl)iron(II) exerts its effects is primarily through its ability to donate and accept electrons. The iron(II) core can be easily oxidized to iron(III), facilitating redox reactions. The pentamethylcyclopentadienyl ligands stabilize the iron center, allowing for controlled electron transfer processes .
Comparación Con Compuestos Similares
Similar Compounds
Ferrocene: The parent compound of bis(pentamethylcyclopentadienyl)iron(II), with hydrogen atoms instead of methyl groups on the cyclopentadienyl rings.
Bis(pentamethylcyclopentadienyl)cobalt(II): Similar structure but with cobalt instead of iron.
Bis(pentamethylcyclopentadienyl)nickel(II): Similar structure but with nickel instead of iron
Uniqueness
Bis(pentamethylcyclopentadienyl)iron(II) is unique due to the presence of methyl groups on the cyclopentadienyl rings, which enhance its stability and electron-donating properties compared to ferrocene. This makes it a more effective reducing agent and a valuable compound for studying redox chemistry .
Propiedades
Número CAS |
12126-50-0 |
---|---|
Fórmula molecular |
C20H30Fe |
Peso molecular |
326.3 g/mol |
Nombre IUPAC |
iron(2+);1,2,3,4,5-pentamethylcyclopenta-1,3-diene |
InChI |
InChI=1S/2C10H15.Fe/c2*1-6-7(2)9(4)10(5)8(6)3;/h2*1-5H3;/q2*-1;+2 |
Clave InChI |
SEYZDJPFVVXSRB-UHFFFAOYSA-N |
SMILES |
C[C-]1[C-]([C-]([C-]([C-]1C)C)C)C.C[C-]1C(=C(C(=C1C)C)C)C.[Fe] |
SMILES canónico |
C[C-]1C(=C(C(=C1C)C)C)C.C[C-]1C(=C(C(=C1C)C)C)C.[Fe+2] |
Origen del producto |
United States |
Q1: How does the use of Bis(pentamethylcyclopentadienyl)iron(II) as a reducing agent compare to ferrocene in reactions with Cu(dmp)22+ (dmp = 2,9-dimethyl-1,10-phenanthroline)?
A1: Research indicates that while both Bis(pentamethylcyclopentadienyl)iron(II) and ferrocene can act as reducing agents for Cu(dmp)22+, their reaction mechanisms differ significantly [1]. While the reduction with ferrocene primarily follows a pathway dependent on the deformation of Cu(dmp)22+, Bis(pentamethylcyclopentadienyl)iron(II), possessing a significantly more negative E° value (500 mV lower than ferrocene [1]), promotes a reaction pathway influenced by the deformation of Cu(dmp)2+ [1]. This difference highlights the impact of the reductant's reduction potential on the reaction pathway and the species involved in the rate-determining steps.
Q2: Can Bis(pentamethylcyclopentadienyl)iron(II) be used to study electrochemical processes at liquid-liquid interfaces?
A2: Yes, Bis(pentamethylcyclopentadienyl)iron(II) has shown promise as a redox probe in studying electron transfer across liquid-liquid interfaces [2]. Its use in this context allows researchers to investigate the kinetics and thermodynamics of charge transfer processes occurring at the interface between immiscible liquids.
- Kano, K., et al. (2002). An Interpretation of Gated Behavior: Kinetic Studies of the Oxidation and Reduction Reactions of Bis(2,9-dimethyl-1,10-phenanthroline)copper(I/II) in Acetonitrile. Bulletin of the Chemical Society of Japan, 75(1), 1-13.
- Reymond, F., et al. (2003). Voltammetric study of electron transfer across the 1,6-dichlorohexane|water interface with the bis(pentamethylcyclopentadienyl)iron(II/III) redox couple. Journal of Electroanalytical Chemistry, 556(1-2), 27-35.
Descargo de responsabilidad e información sobre productos de investigación in vitro
Tenga en cuenta que todos los artículos e información de productos presentados en BenchChem están destinados únicamente con fines informativos. Los productos disponibles para la compra en BenchChem están diseñados específicamente para estudios in vitro, que se realizan fuera de organismos vivos. Los estudios in vitro, derivados del término latino "in vidrio", involucran experimentos realizados en entornos de laboratorio controlados utilizando células o tejidos. Es importante tener en cuenta que estos productos no se clasifican como medicamentos y no han recibido la aprobación de la FDA para la prevención, tratamiento o cura de ninguna condición médica, dolencia o enfermedad. Debemos enfatizar que cualquier forma de introducción corporal de estos productos en humanos o animales está estrictamente prohibida por ley. Es esencial adherirse a estas pautas para garantizar el cumplimiento de los estándares legales y éticos en la investigación y experimentación.