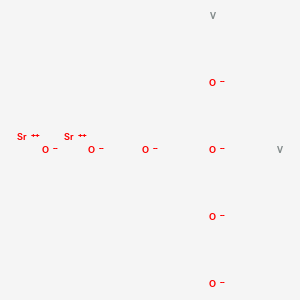
Strontium vanadium oxide (SrV2O6)
- Haga clic en CONSULTA RÁPIDA para recibir una cotización de nuestro equipo de expertos.
- Con productos de calidad a un precio COMPETITIVO, puede centrarse más en su investigación.
Descripción general
Descripción
Strontium vanadium oxide (SrV2O6) is a compound that crystallizes in the orthorhombic Pnma space group . The structure is three-dimensional where Sr2+ is bonded in a 9-coordinate geometry to nine O2- atoms . There are two inequivalent V5+ sites. In the first V5+ site, V5+ is bonded in a 5-coordinate geometry to five O2- atoms .
Synthesis Analysis
The synthesis of SrV2O6 has been achieved through various methods. One such method is the hydrothermal method . The rod-like SrV2O6 was prepared without using any surfactants or templates . The successful formation of rod-like SrV2O6 was confirmed by various analytical and spectroscopic techniques .Molecular Structure Analysis
SrV2O6 has a unique molecular structure. It crystallizes in the orthorhombic Pnma space group . The structure is three-dimensional where Sr2+ is bonded in a 9-coordinate geometry to nine O2- atoms . There are two inequivalent V5+ sites. In the first V5+ site, V5+ is bonded in a 5-coordinate geometry to five O2- atoms .Chemical Reactions Analysis
Vanadium oxides, including SrV2O6, have been known to exhibit extreme sensitivity to external stimuli such as pressure, temperature, or doping . As a result, several vanadium oxides undergo insulator-to-metal phase transition (IMT) accompanied by structural change .Mecanismo De Acción
While the exact mechanism of action for SrV2O6 is not fully understood, strontium-based compounds have been shown to have unique mechanisms. For example, strontium ranelate can slow down the progression of osteoarthritis of the knee. This agent presents an atypical mechanism of action in which it increases deposition of new bone by osteoblasts and, simultaneously, reduces the resorption of bone by osteoclasts .
Direcciones Futuras
The use of vanadium oxide clusters as atomically precise models for bulk metal oxides is a promising area of research . This includes the assembly of vanadium oxide clusters and the consequences of altering the molecular composition of the assembly via organofunctionalization and the incorporation of elemental “dopants” . This could lead to new insights and applications in the future.
Propiedades
IUPAC Name |
distrontium;oxygen(2-);vanadium |
Source
|
---|---|---|
Source | PubChem | |
URL | https://pubchem.ncbi.nlm.nih.gov | |
Description | Data deposited in or computed by PubChem | |
InChI |
InChI=1S/7O.2Sr.2V/q7*-2;2*+2;; |
Source
|
Source | PubChem | |
URL | https://pubchem.ncbi.nlm.nih.gov | |
Description | Data deposited in or computed by PubChem | |
InChI Key |
YMNMFUIJDSASQW-UHFFFAOYSA-N |
Source
|
Source | PubChem | |
URL | https://pubchem.ncbi.nlm.nih.gov | |
Description | Data deposited in or computed by PubChem | |
Canonical SMILES |
[O-2].[O-2].[O-2].[O-2].[O-2].[O-2].[O-2].[V].[V].[Sr+2].[Sr+2] |
Source
|
Source | PubChem | |
URL | https://pubchem.ncbi.nlm.nih.gov | |
Description | Data deposited in or computed by PubChem | |
Molecular Formula |
O7Sr2V2-10 |
Source
|
Source | PubChem | |
URL | https://pubchem.ncbi.nlm.nih.gov | |
Description | Data deposited in or computed by PubChem | |
Molecular Weight |
389.1 g/mol |
Source
|
Source | PubChem | |
URL | https://pubchem.ncbi.nlm.nih.gov | |
Description | Data deposited in or computed by PubChem | |
CAS RN |
13597-58-5 |
Source
|
Record name | Strontium vanadium oxide (SrV2O6) | |
Source | ChemIDplus | |
URL | https://pubchem.ncbi.nlm.nih.gov/substance/?source=chemidplus&sourceid=0013597585 | |
Description | ChemIDplus is a free, web search system that provides access to the structure and nomenclature authority files used for the identification of chemical substances cited in National Library of Medicine (NLM) databases, including the TOXNET system. | |
Record name | Strontium vanadium oxide (SrV2O6) | |
Source | EPA Chemicals under the TSCA | |
URL | https://www.epa.gov/chemicals-under-tsca | |
Description | EPA Chemicals under the Toxic Substances Control Act (TSCA) collection contains information on chemicals and their regulations under TSCA, including non-confidential content from the TSCA Chemical Substance Inventory and Chemical Data Reporting. | |
Record name | Strontium divanadium hexaoxide | |
Source | European Chemicals Agency (ECHA) | |
URL | https://echa.europa.eu/substance-information/-/substanceinfo/100.033.671 | |
Description | The European Chemicals Agency (ECHA) is an agency of the European Union which is the driving force among regulatory authorities in implementing the EU's groundbreaking chemicals legislation for the benefit of human health and the environment as well as for innovation and competitiveness. | |
Explanation | Use of the information, documents and data from the ECHA website is subject to the terms and conditions of this Legal Notice, and subject to other binding limitations provided for under applicable law, the information, documents and data made available on the ECHA website may be reproduced, distributed and/or used, totally or in part, for non-commercial purposes provided that ECHA is acknowledged as the source: "Source: European Chemicals Agency, http://echa.europa.eu/". Such acknowledgement must be included in each copy of the material. ECHA permits and encourages organisations and individuals to create links to the ECHA website under the following cumulative conditions: Links can only be made to webpages that provide a link to the Legal Notice page. | |
Descargo de responsabilidad e información sobre productos de investigación in vitro
Tenga en cuenta que todos los artículos e información de productos presentados en BenchChem están destinados únicamente con fines informativos. Los productos disponibles para la compra en BenchChem están diseñados específicamente para estudios in vitro, que se realizan fuera de organismos vivos. Los estudios in vitro, derivados del término latino "in vidrio", involucran experimentos realizados en entornos de laboratorio controlados utilizando células o tejidos. Es importante tener en cuenta que estos productos no se clasifican como medicamentos y no han recibido la aprobación de la FDA para la prevención, tratamiento o cura de ninguna condición médica, dolencia o enfermedad. Debemos enfatizar que cualquier forma de introducción corporal de estos productos en humanos o animales está estrictamente prohibida por ley. Es esencial adherirse a estas pautas para garantizar el cumplimiento de los estándares legales y éticos en la investigación y experimentación.