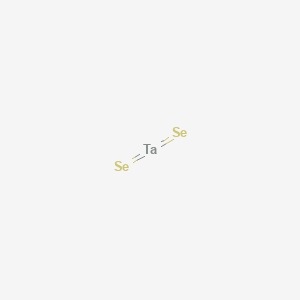
Selenuro de tantalio
Descripción general
Descripción
The hexagonal layered crystal structure of Tantalum diselenide constitutes of Se-Ta-Se layers in various stacking arrangements. The layered structure allows lamination or exfoliation of the layers.
Aplicaciones Científicas De Investigación
Electronic Applications
Charge Density Wave Characteristics
TaSe₂ exhibits charge density wave (CDW) phenomena, which are critical for its electronic applications. The 2H phase of TaSe₂ shows a high CDW transition temperature, making it suitable for devices that exploit these properties. Studies have demonstrated that monolayer 1T-TaSe₂ can exhibit a CDW-Mott phase with a transition temperature of approximately 530 K, indicating its potential for high-temperature applications in electronics .
Hot Electron Injection
The ability of TaSe₂ to generate hot electrons with long lifetimes makes it an excellent candidate for hot electron injection in semiconductor devices. This property is particularly useful in enhancing the performance of photodetectors and other optoelectronic devices . For instance, a vertical photodetector utilizing TaSe₂ demonstrated a responsivity exceeding 10 A/W at 0.1 MHz, showcasing its effectiveness in high-speed applications .
Optoelectronic Applications
Optical Absorption and Emission
TaSe₂ is noted for its strong optical absorption and photoluminescence properties. It can serve as a donor layer for non-radiative resonant energy transfer to other materials, such as molybdenum disulfide (MoS₂). This capability allows for enhanced light emission in heterostructures involving TaSe₂ and other transition metal dichalcogenides . The material's ability to absorb light effectively at wavelengths around 532 nm positions it as a promising candidate for developing new optoelectronic devices.
Saturable Absorbers in Laser Technology
Recent studies have identified TaSe₂ as a potential saturable absorber for pulsed laser applications. Its metallic band structure allows it to function effectively in dual-wavelength Q-switched fiber lasers, achieving pulse widths of approximately 2.95 μs and peak powers up to 64 W . This application highlights TaSe₂'s versatility in photonics and laser technology.
Photonic Devices
Photodetectors
The combination of strong electron–phonon coupling and metallic conductivity makes TaSe₂ an ideal material for photodetectors. Its ability to inject hot carriers efficiently enhances the performance of these devices, allowing them to operate at higher speeds and sensitivities than traditional materials .
Flexible Optoelectronic Devices
Due to its layered structure, TaSe₂ can be integrated into flexible electronic devices. The potential for wafer-scale growth further supports its application in creating flexible "all-2D" optoelectronic systems that can be used in various consumer electronics and wearable technologies .
Research Insights and Case Studies
Mecanismo De Acción
Mode of Action:
TaSe₂ consists of a hexagonal layer of tantalum atoms sandwiched between two layers of selenium atoms. These layers are stacked together via weak van der Waals interactions, forming two-dimensional nanosheets. The electronic structure of TaSe₂ depends on the filling of tantalum’s d orbitals, resulting in metallic behavior . When TaSe₂ interacts with protons during the HER, it facilitates the reduction of protons to form hydrogen gas. This interaction occurs at exposed active sites on the material’s surface.
Biochemical Pathways:
The HER pathway involves the adsorption of protons (H⁺) onto the surface of TaSe₂. The material’s basal-plane sites play a crucial role in accelerating electrode kinetics and enhancing charge transfer. As a result, TaSe₂ optimizes its morphologies during the HER process, leading to improved intrinsic electrocatalytic performance .
Actividad Biológica
Tantalum selenide (TaSe₂) is a transition metal dichalcogenide (TMD) that has garnered attention for its unique properties and potential applications in various fields, including biomedicine. This article explores the biological activity of TaSe₂, focusing on its synthesis, antimicrobial properties, and potential applications in biomedical fields.
Overview of Tantalum Selenide
Tantalum selenide is a layered compound consisting of tantalum and selenium atoms, characterized by its metallic properties and charge density wave (CDW) transitions. The compound exists in different structural phases, primarily 2H and 1T, which exhibit distinct electronic properties. The 2H phase is stable at room temperature and is known for its superconducting behavior under certain conditions .
Synthesis Methods
TaSe₂ can be synthesized using various methods, including:
- Solid-State Synthesis : Involves heating tantalum and selenium in a controlled environment.
- Liquid Phase Exfoliation : This method allows for the production of nanosheets by dispersing bulk TaSe₂ in solvents like ethanol and applying ultrasonic waves to break down the layers .
- Hydrothermal Methods : These techniques can modify the surface properties of TaSe₂, enhancing its biological activity .
Antimicrobial Properties
Recent studies have highlighted the antimicrobial potential of TaSe₂. A comparative study evaluated the efficacy of TaSe₂ against common bacterial strains such as Escherichia coli and Staphylococcus aureus. Molecular docking studies indicated that TaSe₂ interacts with key bacterial proteins involved in cell wall biosynthesis and metabolic pathways, which are crucial for bacterial survival .
Table 1: Antimicrobial Activity of TaSe₂
Bacterial Strain | Minimum Inhibitory Concentration (MIC) | Mechanism of Action |
---|---|---|
E. coli | 50 µg/mL | Inhibition of β-lactamase |
S. aureus | 30 µg/mL | Disruption of fatty acid biosynthesis |
The study demonstrated that TaSe₂ exhibits significant antimicrobial activity, suggesting its potential use as an antibacterial agent in medical applications.
Biocompatibility Studies
In addition to its antimicrobial properties, TaSe₂ has been evaluated for biocompatibility. Research involving cell viability assays (MTT assays) indicated that TaSe₂ does not exhibit cytotoxic effects on human cell lines at certain concentrations. This suggests its suitability for biomedical applications, particularly in implants and drug delivery systems .
Case Studies
Case Study 1: Antibacterial Coatings
A study investigated the application of TaSe₂ as a coating material for medical implants. The coated implants showed reduced bacterial colonization compared to uncoated controls. This highlights the potential of TaSe₂ to prevent infections associated with surgical implants.
Case Study 2: Drug Delivery Systems
Another research focused on using TaSe₂ nanosheets for drug delivery. The nanosheets were functionalized to enhance drug loading capacity and release profiles. The results showed that TaSe₂ could effectively deliver therapeutic agents while maintaining low toxicity to human cells .
Propiedades
IUPAC Name |
bis(selanylidene)tantalum | |
---|---|---|
Source | PubChem | |
URL | https://pubchem.ncbi.nlm.nih.gov | |
Description | Data deposited in or computed by PubChem | |
InChI |
InChI=1S/2Se.Ta | |
Source | PubChem | |
URL | https://pubchem.ncbi.nlm.nih.gov | |
Description | Data deposited in or computed by PubChem | |
InChI Key |
IYJABVNLJXJBTP-UHFFFAOYSA-N | |
Source | PubChem | |
URL | https://pubchem.ncbi.nlm.nih.gov | |
Description | Data deposited in or computed by PubChem | |
Canonical SMILES |
[Se]=[Ta]=[Se] | |
Source | PubChem | |
URL | https://pubchem.ncbi.nlm.nih.gov | |
Description | Data deposited in or computed by PubChem | |
Molecular Formula |
Se2Ta | |
Source | PubChem | |
URL | https://pubchem.ncbi.nlm.nih.gov | |
Description | Data deposited in or computed by PubChem | |
DSSTOX Substance ID |
DTXSID6065210 | |
Record name | Tantalum selenide (TaSe2) | |
Source | EPA DSSTox | |
URL | https://comptox.epa.gov/dashboard/DTXSID6065210 | |
Description | DSSTox provides a high quality public chemistry resource for supporting improved predictive toxicology. | |
Molecular Weight |
338.89 g/mol | |
Source | PubChem | |
URL | https://pubchem.ncbi.nlm.nih.gov | |
Description | Data deposited in or computed by PubChem | |
CAS No. |
12039-55-3 | |
Record name | Tantalum selenide (TaSe2) | |
Source | CAS Common Chemistry | |
URL | https://commonchemistry.cas.org/detail?cas_rn=12039-55-3 | |
Description | CAS Common Chemistry is an open community resource for accessing chemical information. Nearly 500,000 chemical substances from CAS REGISTRY cover areas of community interest, including common and frequently regulated chemicals, and those relevant to high school and undergraduate chemistry classes. This chemical information, curated by our expert scientists, is provided in alignment with our mission as a division of the American Chemical Society. | |
Explanation | The data from CAS Common Chemistry is provided under a CC-BY-NC 4.0 license, unless otherwise stated. | |
Record name | Tantalum selenide (TaSe2) | |
Source | ChemIDplus | |
URL | https://pubchem.ncbi.nlm.nih.gov/substance/?source=chemidplus&sourceid=0012039553 | |
Description | ChemIDplus is a free, web search system that provides access to the structure and nomenclature authority files used for the identification of chemical substances cited in National Library of Medicine (NLM) databases, including the TOXNET system. | |
Record name | Tantalum selenide (TaSe2) | |
Source | EPA Chemicals under the TSCA | |
URL | https://www.epa.gov/chemicals-under-tsca | |
Description | EPA Chemicals under the Toxic Substances Control Act (TSCA) collection contains information on chemicals and their regulations under TSCA, including non-confidential content from the TSCA Chemical Substance Inventory and Chemical Data Reporting. | |
Record name | Tantalum selenide (TaSe2) | |
Source | EPA DSSTox | |
URL | https://comptox.epa.gov/dashboard/DTXSID6065210 | |
Description | DSSTox provides a high quality public chemistry resource for supporting improved predictive toxicology. | |
Record name | Tantalum diselenide | |
Source | European Chemicals Agency (ECHA) | |
URL | https://echa.europa.eu/substance-information/-/substanceinfo/100.031.713 | |
Description | The European Chemicals Agency (ECHA) is an agency of the European Union which is the driving force among regulatory authorities in implementing the EU's groundbreaking chemicals legislation for the benefit of human health and the environment as well as for innovation and competitiveness. | |
Explanation | Use of the information, documents and data from the ECHA website is subject to the terms and conditions of this Legal Notice, and subject to other binding limitations provided for under applicable law, the information, documents and data made available on the ECHA website may be reproduced, distributed and/or used, totally or in part, for non-commercial purposes provided that ECHA is acknowledged as the source: "Source: European Chemicals Agency, http://echa.europa.eu/". Such acknowledgement must be included in each copy of the material. ECHA permits and encourages organisations and individuals to create links to the ECHA website under the following cumulative conditions: Links can only be made to webpages that provide a link to the Legal Notice page. | |
Descargo de responsabilidad e información sobre productos de investigación in vitro
Tenga en cuenta que todos los artículos e información de productos presentados en BenchChem están destinados únicamente con fines informativos. Los productos disponibles para la compra en BenchChem están diseñados específicamente para estudios in vitro, que se realizan fuera de organismos vivos. Los estudios in vitro, derivados del término latino "in vidrio", involucran experimentos realizados en entornos de laboratorio controlados utilizando células o tejidos. Es importante tener en cuenta que estos productos no se clasifican como medicamentos y no han recibido la aprobación de la FDA para la prevención, tratamiento o cura de ninguna condición médica, dolencia o enfermedad. Debemos enfatizar que cualquier forma de introducción corporal de estos productos en humanos o animales está estrictamente prohibida por ley. Es esencial adherirse a estas pautas para garantizar el cumplimiento de los estándares legales y éticos en la investigación y experimentación.