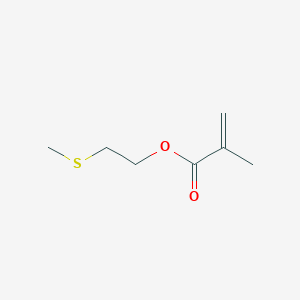
2-(Methylthio)ethyl methacrylate
Descripción general
Descripción
2-(Methylthio)ethyl methacrylate
Mecanismo De Acción
Target of Action
2-(Methylthio)ethyl methacrylate (2-MEMA) is a monomer that is primarily used in the synthesis of polymers . The primary targets of 2-MEMA are the polymer chains where it gets incorporated during the polymerization process .
Mode of Action
The mode of action of 2-MEMA involves its incorporation into polymer chains through a process known as polymerization . During this process, the double bond in the methacrylate group of 2-MEMA opens up and forms a bond with the growing polymer chain . This results in the formation of a polymer that has the 2-(methylthio)ethyl group pendant from the polymer backbone .
Biochemical Pathways
The polymers synthesized from 2-mema can exhibit various properties depending on their structure and composition . These properties can influence the behavior of the polymers in biological systems, such as their biocompatibility, biodegradability, and ability to interact with cells and tissues .
Pharmacokinetics
The pharmacokinetics of the resulting polymers would depend on factors such as their size, chemical composition, and the presence of any functional groups .
Result of Action
The primary result of the action of 2-MEMA is the formation of polymers with specific properties conferred by the 2-(methylthio)ethyl group . These polymers can be designed to have a variety of uses, such as in the development of new materials, drug delivery systems, and biomedical devices .
Análisis Bioquímico
Biochemical Properties
It is known that it can undergo polymerization under free-radical conditions .
Cellular Effects
It has been reported that polymers derived from this compound can be used in cryopreservation . These polymers do not modulate the frozen components during cryopreservation . Long-term exposure of the polymers was not tolerated by cells, but shorter incubation times, which are relevant for cryopreservation, were tolerated .
Molecular Mechanism
It is known that it can undergo polymerization under free-radical conditions .
Temporal Effects in Laboratory Settings
It has been reported that polymers derived from this compound can be used in cryopreservation . These polymers do not modulate the frozen components during cryopreservation .
Actividad Biológica
2-(Methylthio)ethyl methacrylate (MTEMA) is a compound that has garnered attention in various fields, particularly in polymer science and biochemistry. Its unique structure allows it to serve as a versatile monomer for synthesizing polymers with specific biological activities. This article delves into the biological activity of MTEMA, exploring its mechanisms of action, applications, and relevant research findings.
Chemical Structure and Properties
MTEMA is characterized by the presence of a methylthio group attached to an ethyl methacrylate backbone. This configuration imparts distinctive chemical properties that facilitate its use in various applications, including drug delivery systems and antimicrobial agents.
- Chemical Formula : C₇H₁₄O₂S
- CAS Number : 14216-23-0
Mechanisms of Biological Activity
The biological activity of MTEMA can be attributed to several mechanisms:
- Polymerization-Induced Self-Assembly : MTEMA can undergo polymerization to form self-assembling structures that respond to environmental stimuli. This property is crucial for developing smart materials in drug delivery systems where controlled release is desired .
- Antimicrobial Properties : Research indicates that MTEMA derivatives exhibit significant antimicrobial activity. For instance, studies have shown that polymers synthesized from MTEMA can inhibit the growth of various bacteria, making them suitable for medical applications such as wound dressings .
- Adhesion Promoter : MTEMA has been identified as an effective adhesion promoter in various substrates, enhancing the bonding strength of coatings and composites .
1. Antimicrobial Activity
A study investigated the antimicrobial efficacy of MTEMA-based polymers against Staphylococcus aureus and Escherichia coli. The results demonstrated that polymers containing MTEMA exhibited a substantial reduction in bacterial viability, suggesting potential applications in antimicrobial coatings and medical devices.
Polymer Composition | Bacterial Strain | Viability Reduction (%) |
---|---|---|
MTEMA + PEG | S. aureus | 95 |
MTEMA + PEG | E. coli | 90 |
2. Drug Delivery Systems
Another study focused on the use of MTEMA in drug delivery applications. The polymer formed from MTEMA was tested for its ability to encapsulate and release therapeutic agents in a controlled manner. The findings indicated that the release profile could be modulated by adjusting the degree of crosslinking in the polymer network.
Drug | Encapsulation Efficiency (%) | Release Rate (mg/h) |
---|---|---|
Doxorubicin | 85 | 0.5 |
Paclitaxel | 78 | 0.3 |
Propiedades
IUPAC Name |
2-methylsulfanylethyl 2-methylprop-2-enoate | |
---|---|---|
Source | PubChem | |
URL | https://pubchem.ncbi.nlm.nih.gov | |
Description | Data deposited in or computed by PubChem | |
InChI |
InChI=1S/C7H12O2S/c1-6(2)7(8)9-4-5-10-3/h1,4-5H2,2-3H3 | |
Source | PubChem | |
URL | https://pubchem.ncbi.nlm.nih.gov | |
Description | Data deposited in or computed by PubChem | |
InChI Key |
MLGMNVSTCSILMV-UHFFFAOYSA-N | |
Source | PubChem | |
URL | https://pubchem.ncbi.nlm.nih.gov | |
Description | Data deposited in or computed by PubChem | |
Canonical SMILES |
CC(=C)C(=O)OCCSC | |
Source | PubChem | |
URL | https://pubchem.ncbi.nlm.nih.gov | |
Description | Data deposited in or computed by PubChem | |
Molecular Formula |
C7H12O2S | |
Source | PubChem | |
URL | https://pubchem.ncbi.nlm.nih.gov | |
Description | Data deposited in or computed by PubChem | |
DSSTOX Substance ID |
DTXSID50393393 | |
Record name | 2-(Methylthio)ethyl methacrylate | |
Source | EPA DSSTox | |
URL | https://comptox.epa.gov/dashboard/DTXSID50393393 | |
Description | DSSTox provides a high quality public chemistry resource for supporting improved predictive toxicology. | |
Molecular Weight |
160.24 g/mol | |
Source | PubChem | |
URL | https://pubchem.ncbi.nlm.nih.gov | |
Description | Data deposited in or computed by PubChem | |
CAS No. |
14216-23-0 | |
Record name | 2-(Methylthio)ethyl methacrylate | |
Source | EPA DSSTox | |
URL | https://comptox.epa.gov/dashboard/DTXSID50393393 | |
Description | DSSTox provides a high quality public chemistry resource for supporting improved predictive toxicology. | |
Retrosynthesis Analysis
AI-Powered Synthesis Planning: Our tool employs the Template_relevance Pistachio, Template_relevance Bkms_metabolic, Template_relevance Pistachio_ringbreaker, Template_relevance Reaxys, Template_relevance Reaxys_biocatalysis model, leveraging a vast database of chemical reactions to predict feasible synthetic routes.
One-Step Synthesis Focus: Specifically designed for one-step synthesis, it provides concise and direct routes for your target compounds, streamlining the synthesis process.
Accurate Predictions: Utilizing the extensive PISTACHIO, BKMS_METABOLIC, PISTACHIO_RINGBREAKER, REAXYS, REAXYS_BIOCATALYSIS database, our tool offers high-accuracy predictions, reflecting the latest in chemical research and data.
Strategy Settings
Precursor scoring | Relevance Heuristic |
---|---|
Min. plausibility | 0.01 |
Model | Template_relevance |
Template Set | Pistachio/Bkms_metabolic/Pistachio_ringbreaker/Reaxys/Reaxys_biocatalysis |
Top-N result to add to graph | 6 |
Feasible Synthetic Routes
Q1: What makes MTEMA particularly interesting for material design?
A1: MTEMA's thioether group offers unique reactivity and can be utilized for stimuli-responsive materials. For example, research has shown that MTEMA can be incorporated into polymers and subsequently oxidized to form sulfoxide groups []. This oxidation process can significantly impact the polymer's properties, such as its cytotoxicity []. Additionally, the thioether group allows for polymerization-induced self-assembly (PISA) when used with specific macro-CTAs like poly(oligo(ethylene glycol) methyl ether methacrylate) (POEGMA) []. This process generates nanoparticles that can be disassembled upon exposure to visible light due to the oxidation of the thioether group by singlet oxygen generated by encapsulated photosensitizers like ZnTPP [].
Q2: Can MTEMA be used to create functional surfaces?
A2: Yes, MTEMA can be copolymerized with other monomers to create surfaces with tailored properties. For instance, researchers have successfully incorporated MTEMA into terpolymers containing hydrophilic and hydrophobic components []. This specific terpolymer was then used as a platform for the in-situ polymerization of phospholipids, resulting in a stable, biomimetic surface [].
Q3: Does the incorporation of MTEMA affect the electrochemical properties of polymers?
A3: Yes, incorporating MTEMA into polymers with electroactive groups like ferrocene can influence their electrochemical behavior. Studies have demonstrated that copolymers of vinylferrocene and MTEMA exhibit enhanced stability when used to modify gold electrodes compared to poly(vinylferrocene) alone []. This improved stability is attributed to the presence of the thioether groups within the copolymer structure [].
Q4: Are there applications of MTEMA in metal extraction?
A4: Yes, MTEMA-containing polymers show promise for metal extraction applications. Research has shown that poly(N-isopropylacrylamide-co-MTEMA) gels can effectively extract gold(III) ions from solutions []. The thioether groups in MTEMA play a crucial role in binding the gold ions, while the thermosensitive nature of the copolymer allows for the controlled uptake and release of the metal [].
Q5: Are there any limitations or challenges associated with using MTEMA?
A5: While MTEMA offers various advantages, some challenges need to be considered. For instance, the oxidation of the thioether group to sulfoxide, while useful for some applications, can also lead to increased cytotoxicity in certain polymers []. This highlights the need for precise control over the oxidation process to tailor the material properties for specific applications.
Descargo de responsabilidad e información sobre productos de investigación in vitro
Tenga en cuenta que todos los artículos e información de productos presentados en BenchChem están destinados únicamente con fines informativos. Los productos disponibles para la compra en BenchChem están diseñados específicamente para estudios in vitro, que se realizan fuera de organismos vivos. Los estudios in vitro, derivados del término latino "in vidrio", involucran experimentos realizados en entornos de laboratorio controlados utilizando células o tejidos. Es importante tener en cuenta que estos productos no se clasifican como medicamentos y no han recibido la aprobación de la FDA para la prevención, tratamiento o cura de ninguna condición médica, dolencia o enfermedad. Debemos enfatizar que cualquier forma de introducción corporal de estos productos en humanos o animales está estrictamente prohibida por ley. Es esencial adherirse a estas pautas para garantizar el cumplimiento de los estándares legales y éticos en la investigación y experimentación.