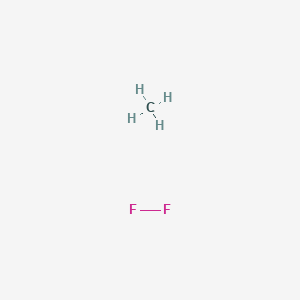
Carbono fluorado
Descripción general
Descripción
Fluorine, compd. with graphite is a useful research compound. Its molecular formula is CH4F2 and its molecular weight is 54.039 g/mol. The purity is usually 95%.
BenchChem offers high-quality Fluorine, compd. with graphite suitable for many research applications. Different packaging options are available to accommodate customers' requirements. Please inquire for more information about Fluorine, compd. with graphite including the price, delivery time, and more detailed information at info@benchchem.com.
Aplicaciones Científicas De Investigación
Chemical Properties and Structure
Fluorinated graphite is characterized by the substitution of carbon atoms in graphite with fluorine atoms, resulting in various structural modifications. The bonding between carbon and fluorine can be ionic or covalent, which influences the material's electrical conductivity and thermal properties. The ratio of these bonds can vary based on fluorination conditions such as temperature and the presence of catalysts .
Lubricants
Fluorinated graphite exhibits low friction coefficients, making it an excellent candidate for lubricants. The presence of fluorine reduces the energy required for sheet cleavage in graphite, leading to improved lubrication properties. Studies have shown that fluorinated graphite outperforms non-fluorinated counterparts in tribological applications, providing superior wear resistance and endurance life .
Battery Technologies
Fluorinated carbon materials are being researched for use in battery cathodes due to their enhanced electrical conductivity. The ionic bonds formed between fluorine and carbon increase hole carriers, thereby improving charge transport properties . This characteristic is particularly advantageous for lithium-ion batteries where efficient charge transfer is crucial.
Water Repellency
The hydrophobic nature of fluorinated graphite makes it suitable for applications requiring water repellency. Fluorinated surfaces exhibit reduced wettability, which can be beneficial in coatings and materials designed to resist moisture absorption .
Magnetic Properties
Recent studies have investigated the magnetic properties of fluorinated graphene structures, revealing phenomena such as spin-half Curie paramagnetism and ferromagnetic-like responses under specific conditions. These properties open avenues for applications in magnetic materials and devices .
Tribological Performance Evaluation
A comparative study on the tribological properties of fluorinated graphite versus non-fluorinated graphite demonstrated significant improvements in wear resistance and friction reduction. The endurance life of fluorinated graphite was found to be up to five times greater than that of non-fluorinated materials, highlighting its effectiveness as a lubricant .
Material Type | Wear Rate (mg/km) | Endurance Life (cycles) |
---|---|---|
Non-Fluorinated Graphite | 150 | 500 |
Fluorinated Graphite | 30 | 2500 |
Electrical Conductivity in Battery Applications
Research into the use of fluorinated graphite as a cathode material in lithium-ion batteries showed that varying the degree of fluorination could tune the electrical conductivity significantly. A study reported a conductivity increase by over 200% with optimized fluorination conditions .
Mecanismo De Acción
Target of Action
Fluorinated carbon (CFx) is a thriving member of the carbonaceous derivative family . It primarily targets energy conversion and storage devices, biomedicines, gas sensors, electronic devices, and microwave absorption devices . The compound’s primary targets are the structures and systems within these devices where it interacts with other elements and compounds to produce desired effects .
Mode of Action
Fluorinated carbon contains various types of C-F bonds including ionic, semi-ionic, and covalent C-F, C-F2, C-F3 bonds with tunable F/C ratios . The controllable designing of C-F bonding and F/C ratios play a key role in optimizing the properties of fluorinated carbon materials . The C-F bonds are formed based on the fluorination reactions between carbon materials and fluorinating agents .
Biochemical Pathways
The synthesis methods of fluorinated carbon can be mainly classified into direct gas fluorination, indirect fluorination, and plasma-assisted fluorination . The C-F bonds and the F/C ratios deeply depend on the raw carbon materials and fluorination conditions including fluorinating agents, temperature, and reaction time . Thus, the key point of fluorinated carbon materials is mainly about the design of carbon materials and the optimization of fluoridation conditions .
Pharmacokinetics
Selective F substitution onto a therapeutic or diagnostic drug candidate can enhance several pharmacokinetic and physicochemical properties such as metabolic stability and membrane permeation . The increased binding ability of fluorinated drug target proteins has also been reported in some cases .
Result of Action
Fluorinated carbon exhibits various excellent properties of chemically stable, tunable bandgap, good thermal conductivity and stability, and super-hydrophobic due to its unique structures and polar C-F bonding . It has been extensively served as a promising positive electrode material for lithium primary batteries due to its high energy density . Furthermore, the electrochemical discharge mechanism based on conversion reaction was further uncovered by applying XPS, XRD, SEM, and EDS elemental analysis characterization techniques .
Action Environment
The action of fluorinated carbon is influenced by environmental factors such as temperature and pressure. For instance, CF0.85 exhibits excellent electrochemical property with delivering a remarkable discharge capacity of 503 mA h g−1 and energy density of 388 W h kg−1 at a current rate of 30 mA g−1 under a temperature of 25 °C . It also exhibits decent temperature performance with discharge capacities of 550 mA h g−1 at 50 °C and 460 mA h g−1 at 0 °C under current density of 30 mA g−1 .
Análisis Bioquímico
Biochemical Properties
Fluorinated carbon contains various types of C-F bonds including ionic, semi-ionic, and covalent C-F, C-F2, C-F3 bonds with tunable F/C ratios . The controllable designing of C-F bonding and F/C ratios plays a key role in optimizing the properties of fluorinated carbon materials . The strength of the carbon-fluorine bond conveys stability to fluorinated drugs .
Cellular Effects
They are strongly resistant to biological and chemical degradation .
Molecular Mechanism
The C-F bonds in fluorinated carbon are formed based on the fluorination reactions between carbon materials and fluorinating agents . Because the F atom has extremely strong electronegativity, fluorinated carbon involves various C-F bonding characteristics of ionic, semi-ionic, and covalent bonds .
Actividad Biológica
Fluorine compounds, particularly those involving graphite, have garnered significant attention in the fields of materials science and medicinal chemistry due to their unique properties and biological activities. This article explores the biological activity of "Fluorine, compd. with graphite," focusing on its chemical interactions, potential therapeutic applications, and structural characteristics.
Overview of Fluorinated Graphite
Fluorinated graphite is a derivative of graphite where fluorine atoms are chemically bonded to carbon atoms. This modification alters the electronic properties and chemical reactivity of graphite, leading to enhanced performance in various applications, including drug delivery systems and imaging agents.
The introduction of fluorine atoms into graphite significantly changes its structural properties. The bonding characteristics between carbon and fluorine can vary based on the degree of fluorination. Research has shown that fluorination can lead to both ionic and covalent bonding configurations, which affects the material's stability and reactivity.
Table 1: Bond Lengths in Fluorinated Graphite
Fluorination (%) | C-CF Bond Length (Å) | C-C Bond Length (Å) | C-F Bond Length (Å) |
---|---|---|---|
0 | 1.422 | 1.422 | - |
25 | 1.504 | 1.399 | 1.464 |
50 | 1.561 | 1.552 | 1.376 |
100 | 1.578 | 1.380 | - |
This table illustrates how bond lengths change with varying degrees of fluorination, indicating a shift in electronic properties due to the presence of electronegative fluorine atoms .
Biological Activity and Therapeutic Applications
The biological activity of fluorinated compounds, including those derived from graphite, stems from their ability to interact with biological systems at the molecular level. The incorporation of fluorine can enhance drug potency, improve metabolic stability, and alter pharmacokinetics.
- Metabolic Stability : Fluorinated compounds exhibit reduced metabolic oxidation rates due to the blocking effect of fluorine on metabolic pathways. This is particularly relevant for drugs that require prolonged action within the body .
- Lipophilicity Modulation : The presence of fluorine increases lipophilicity, which enhances absorption and distribution in biological systems. This property is crucial for drugs targeting specific tissues or organs .
- Receptor Binding : Fluorinated compounds can improve binding affinity to biological receptors by fitting more effectively into receptor sites, thus enhancing therapeutic effects .
Case Studies
Several studies have demonstrated the potential applications of fluorinated graphite compounds in medicine:
- Drug Delivery Systems : Research has indicated that fluorinated graphite can be used as a carrier for targeted drug delivery due to its ability to encapsulate therapeutic agents while protecting them from metabolic degradation .
- Imaging Agents : Fluorinated compounds are increasingly used in medical imaging techniques such as PET scans due to their favorable chemical properties that enhance imaging contrast .
Propiedades
IUPAC Name |
methane;molecular fluorine | |
---|---|---|
Source | PubChem | |
URL | https://pubchem.ncbi.nlm.nih.gov | |
Description | Data deposited in or computed by PubChem | |
InChI |
InChI=1S/CH4.F2/c;1-2/h1H4; | |
Source | PubChem | |
URL | https://pubchem.ncbi.nlm.nih.gov | |
Description | Data deposited in or computed by PubChem | |
InChI Key |
QLOAVXSYZAJECW-UHFFFAOYSA-N | |
Source | PubChem | |
URL | https://pubchem.ncbi.nlm.nih.gov | |
Description | Data deposited in or computed by PubChem | |
Canonical SMILES |
C.FF | |
Source | PubChem | |
URL | https://pubchem.ncbi.nlm.nih.gov | |
Description | Data deposited in or computed by PubChem | |
Molecular Formula |
CH4F2 | |
Source | PubChem | |
URL | https://pubchem.ncbi.nlm.nih.gov | |
Description | Data deposited in or computed by PubChem | |
Molecular Weight |
54.039 g/mol | |
Source | PubChem | |
URL | https://pubchem.ncbi.nlm.nih.gov | |
Description | Data deposited in or computed by PubChem | |
Physical Description |
Poly(carbon monofluoride): Gray-white solid; Poly(dicarbon monofluoride): Dark gray-black solid; [Merck Index] 27% F minimum: Black odorless powder; 62% F minimum: White odorless powder; [Alfa Aesar MSDS] | |
Record name | Graphite fluoride | |
Source | Haz-Map, Information on Hazardous Chemicals and Occupational Diseases | |
URL | https://haz-map.com/Agents/20977 | |
Description | Haz-Map® is an occupational health database designed for health and safety professionals and for consumers seeking information about the adverse effects of workplace exposures to chemical and biological agents. | |
Explanation | Copyright (c) 2022 Haz-Map(R). All rights reserved. Unless otherwise indicated, all materials from Haz-Map are copyrighted by Haz-Map(R). No part of these materials, either text or image may be used for any purpose other than for personal use. Therefore, reproduction, modification, storage in a retrieval system or retransmission, in any form or by any means, electronic, mechanical or otherwise, for reasons other than personal use, is strictly prohibited without prior written permission. | |
CAS No. |
11113-63-6 | |
Record name | Graphite fluoride | |
Source | ChemIDplus | |
URL | https://pubchem.ncbi.nlm.nih.gov/substance/?source=chemidplus&sourceid=0011113636 | |
Description | ChemIDplus is a free, web search system that provides access to the structure and nomenclature authority files used for the identification of chemical substances cited in National Library of Medicine (NLM) databases, including the TOXNET system. | |
Record name | Fluorine, compd. with graphite (1:?) | |
Source | EPA Chemicals under the TSCA | |
URL | https://www.epa.gov/chemicals-under-tsca | |
Description | EPA Chemicals under the Toxic Substances Control Act (TSCA) collection contains information on chemicals and their regulations under TSCA, including non-confidential content from the TSCA Chemical Substance Inventory and Chemical Data Reporting. | |
Record name | Fluorine, compound with graphite | |
Source | European Chemicals Agency (ECHA) | |
URL | https://echa.europa.eu/substance-information/-/substanceinfo/100.031.210 | |
Description | The European Chemicals Agency (ECHA) is an agency of the European Union which is the driving force among regulatory authorities in implementing the EU's groundbreaking chemicals legislation for the benefit of human health and the environment as well as for innovation and competitiveness. | |
Explanation | Use of the information, documents and data from the ECHA website is subject to the terms and conditions of this Legal Notice, and subject to other binding limitations provided for under applicable law, the information, documents and data made available on the ECHA website may be reproduced, distributed and/or used, totally or in part, for non-commercial purposes provided that ECHA is acknowledged as the source: "Source: European Chemicals Agency, http://echa.europa.eu/". Such acknowledgement must be included in each copy of the material. ECHA permits and encourages organisations and individuals to create links to the ECHA website under the following cumulative conditions: Links can only be made to webpages that provide a link to the Legal Notice page. | |
ANone: Fluorinated carbon doesn't have a single, fixed molecular formula or weight. Its composition is represented as CFx, where 'x' denotes the fluorine-to-carbon ratio, which can vary significantly depending on the synthesis method and conditions. [] This ratio significantly influences the material's properties and applications. []
ANone: Several spectroscopic techniques are employed, including:
- Infrared (IR) Spectroscopy: IR spectroscopy helps determine the fluorine-to-carbon ratio by analyzing the characteristic peaks of C-F bonds. [] This method aids in quantitatively analyzing fluorine content in fluorinated carbon materials. []
- X-ray Photoelectron Spectroscopy (XPS): XPS provides information about the chemical states of carbon and fluorine atoms in the material, revealing the types of C-F bonds present (ionic, semi-ionic, covalent) and their relative abundance. [, , ]
- Raman Spectroscopy: This technique helps analyze the structural changes in the carbon material upon fluorination, providing insights into the degree of graphitization and defects. [, ]
- Solid-State 13C Nuclear Magnetic Resonance (NMR): This method allows for the quantification of unfluorinated carbon within the fluorinated carbon material, which is crucial for understanding its conductivity. []
ANone: Fluorine content significantly impacts properties like:
- Hydrophobicity: As fluorine content increases, the material becomes more hydrophobic due to the low surface energy of C-F bonds. [, ]
- Thermal Stability: Fluorinated carbon exhibits high thermal stability, even at temperatures up to 400°C, making it suitable for high-temperature applications. []
- Electrochemical Performance: The fluorine content and the type of C-F bonds strongly influence the electrochemical performance of fluorinated carbon in batteries. [, , ]
ANone: Fluorinated carbon black enhances the electrical properties of PVDF composites, leading to a high positive temperature coefficient (PTC) intensity and low negative temperature coefficient (NTC) effect. [] The fluorine content in carbon black influences the dispersion and electrical properties of these composites. []
ANone: Fluorination can create weak acid sites on the carbon surface, enabling it to catalyze reactions that proceed through the formation of carbenium ions. [] For example, fluorinated carbon has been explored as a catalyst support for platinum in the reaction of nitrogen oxide (NO) with ammonia (NH3). []
ANone: Both catalytic activity and selectivity to nitrogen (N2) formation vary with fluorine content. Research shows that activity and selectivity increased with increasing fluorine content up to 28% but decreased when the fluorine content reached 65%. []
ANone: Computational techniques provide valuable insights into fluorinated carbon:* Density Functional Theory (DFT): DFT calculations help understand the electronic structure, bonding characteristics, and electrochemical behavior of fluorinated carbon. [, , ] * Molecular Dynamics (MD) Simulations: MD simulations are used to investigate the interfacial strength between fluorinated carbon and other materials, such as in carbon fiber-reinforced composites. []
ANone: The length of the fluorinated carbon chain plays a crucial role in the bioaccumulation of perfluorinated acids (PFCAs), a group of chemicals related to fluorinated carbon. [, ] PFCAs with seven or fewer fluorinated carbons are not considered bioaccumulative, while those with longer chains may pose a higher risk. [, ]
ANone: Fluorination generally enhances the chemical stability of carbon materials, making them more resistant to oxidation, degradation, and solvents. [] This stability makes fluorinated carbon suitable for applications in harsh environments. []
ANone: While fluorinated carbon itself might have limited environmental impact, some fluorinated compounds, particularly perfluorinated substances like PFCAs, raise concerns due to their persistence, bioaccumulation potential, and potential toxicity. [, , ]
ANone: Studies investigating the biodegradation of fluorotelomer alcohols (FTOHs), which can be breakdown products of some fluorinated materials, have shown that microorganisms can break down perfluorinated carbon chains under specific conditions. [] This research suggests the potential for bioremediation of certain fluorinated compounds. []
ANone: Fluorinated carbon is being explored for various applications, including:
- Lithium Batteries: Fluorinated carbon is a promising cathode material for high-energy-density lithium primary batteries due to its high theoretical capacity and wide operating temperature range. [, , , , , ]
- Water Management in Fuel Cells: Superhydrophobic fluorinated carbon is being investigated as a component in microporous layers of fuel cells to enhance water management and oxygen diffusion. []
- Biomedical Applications: Nano-sized fluorinated carbon materials show potential in drug delivery, bioimaging, and cancer therapy due to their unique properties and biocompatibility. [, , ]
- Electronic Devices: Fluorinated carbon's tunable electrical properties and high thermal stability make it suitable for use in electronic devices, such as transistors and sensors. [, ]
Descargo de responsabilidad e información sobre productos de investigación in vitro
Tenga en cuenta que todos los artículos e información de productos presentados en BenchChem están destinados únicamente con fines informativos. Los productos disponibles para la compra en BenchChem están diseñados específicamente para estudios in vitro, que se realizan fuera de organismos vivos. Los estudios in vitro, derivados del término latino "in vidrio", involucran experimentos realizados en entornos de laboratorio controlados utilizando células o tejidos. Es importante tener en cuenta que estos productos no se clasifican como medicamentos y no han recibido la aprobación de la FDA para la prevención, tratamiento o cura de ninguna condición médica, dolencia o enfermedad. Debemos enfatizar que cualquier forma de introducción corporal de estos productos en humanos o animales está estrictamente prohibida por ley. Es esencial adherirse a estas pautas para garantizar el cumplimiento de los estándares legales y éticos en la investigación y experimentación.